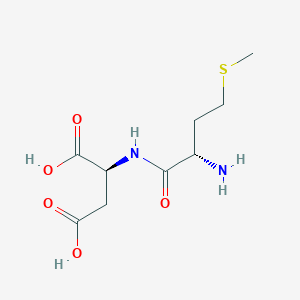
H-Met-Asp-OH
Overview
Description
Mechanism of Action
Methionyl-aspartic acid, also known as H-Met-Asp-OH or Met-Asp, is a dipeptide composed of the amino acids methionine and aspartic acid . This compound plays a role in various biochemical processes, and its mechanism of action can be understood through the following aspects:
Mode of Action
As a dipeptide, it may be involved in protein synthesis, serving as a building block for larger polypeptides . It could also potentially be involved in signaling pathways, given the role of peptides in cell communication.
Biochemical Pathways
Methionyl-aspartic acid is part of the aspartate family of amino acids. The aspartate pathway leads to the synthesis of four key essential amino acids: lysine, methionine, threonine, and isoleucine . Methionine, in particular, has three main functions: it is used in protein synthesis, serves as a methyl donor in transmethylation reactions, and acts as an aminopropyl donor in the synthesis of polyamines .
Preparation Methods
Synthetic Routes and Reaction Conditions
The synthesis of Methionyl-Aspartic Acid typically involves the coupling of L-methionine and L-aspartic acid residues. This can be achieved through standard peptide synthesis techniques, such as solid-phase peptide synthesis (SPPS) or solution-phase peptide synthesis. The reaction conditions often include the use of coupling reagents like dicyclohexylcarbodiimide (DCC) and N-hydroxysuccinimide (NHS) to facilitate the formation of the peptide bond .
Industrial Production Methods
In an industrial setting, the production of Methionyl-Aspartic Acid may involve large-scale peptide synthesis using automated synthesizers. These methods ensure high purity and yield of the desired dipeptide. The process may also include purification steps such as high-performance liquid chromatography (HPLC) to remove any impurities .
Chemical Reactions Analysis
Types of Reactions
Methionyl-Aspartic Acid can undergo various chemical reactions, including:
Oxidation: The methionine residue can be oxidized to methionine sulfoxide or methionine sulfone under oxidative conditions.
Reduction: Reduction reactions can revert oxidized methionine back to its original form.
Substitution: The aspartic acid residue can participate in substitution reactions, particularly at its carboxyl group.
Common Reagents and Conditions
Oxidation: Hydrogen peroxide or other oxidizing agents can be used.
Reduction: Reducing agents like dithiothreitol (DTT) or tris(2-carboxyethyl)phosphine (TCEP) are commonly employed.
Substitution: Various nucleophiles can be used to substitute the carboxyl group of aspartic acid.
Major Products
Oxidation: Methionine sulfoxide, methionine sulfone.
Reduction: Methionine.
Substitution: Derivatives of Methionyl-Aspartic Acid with modified carboxyl groups.
Scientific Research Applications
Methionyl-Aspartic Acid has a wide range of applications in scientific research:
Chemistry: Used as a model compound in peptide synthesis studies and to understand peptide bond formation.
Biology: Investigated for its role in protein structure and function, particularly in the study of protein folding and stability.
Medicine: Explored for its potential therapeutic applications, including as a component of peptide-based drugs.
Industry: Utilized in the production of bioactive peptides and as a building block for more complex peptide structures
Comparison with Similar Compounds
Similar Compounds
Methionyl-Glutamic Acid: Similar to Methionyl-Aspartic Acid but contains glutamic acid instead of aspartic acid.
Methionyl-Serine: Contains serine instead of aspartic acid.
Aspartyl-Phenylalanine: Contains phenylalanine instead of methionine.
Uniqueness
Methionyl-Aspartic Acid is unique due to the presence of both methionine and aspartic acid residues, which confer distinct chemical and biological properties. The methionine residue’s susceptibility to oxidation and the aspartic acid residue’s ability to participate in various chemical reactions make this dipeptide particularly versatile in research and industrial applications .
Properties
IUPAC Name |
(2S)-2-[[(2S)-2-amino-4-methylsulfanylbutanoyl]amino]butanedioic acid | |
---|---|---|
Source | PubChem | |
URL | https://pubchem.ncbi.nlm.nih.gov | |
Description | Data deposited in or computed by PubChem | |
InChI |
InChI=1S/C9H16N2O5S/c1-17-3-2-5(10)8(14)11-6(9(15)16)4-7(12)13/h5-6H,2-4,10H2,1H3,(H,11,14)(H,12,13)(H,15,16)/t5-,6-/m0/s1 | |
Source | PubChem | |
URL | https://pubchem.ncbi.nlm.nih.gov | |
Description | Data deposited in or computed by PubChem | |
InChI Key |
QTZXSYBVOSXBEJ-WDSKDSINSA-N | |
Source | PubChem | |
URL | https://pubchem.ncbi.nlm.nih.gov | |
Description | Data deposited in or computed by PubChem | |
Canonical SMILES |
CSCCC(C(=O)NC(CC(=O)O)C(=O)O)N | |
Source | PubChem | |
URL | https://pubchem.ncbi.nlm.nih.gov | |
Description | Data deposited in or computed by PubChem | |
Isomeric SMILES |
CSCC[C@@H](C(=O)N[C@@H](CC(=O)O)C(=O)O)N | |
Source | PubChem | |
URL | https://pubchem.ncbi.nlm.nih.gov | |
Description | Data deposited in or computed by PubChem | |
Molecular Formula |
C9H16N2O5S | |
Source | PubChem | |
URL | https://pubchem.ncbi.nlm.nih.gov | |
Description | Data deposited in or computed by PubChem | |
Molecular Weight |
264.30 g/mol | |
Source | PubChem | |
URL | https://pubchem.ncbi.nlm.nih.gov | |
Description | Data deposited in or computed by PubChem | |
Retrosynthesis Analysis
AI-Powered Synthesis Planning: Our tool employs the Template_relevance Pistachio, Template_relevance Bkms_metabolic, Template_relevance Pistachio_ringbreaker, Template_relevance Reaxys, Template_relevance Reaxys_biocatalysis model, leveraging a vast database of chemical reactions to predict feasible synthetic routes.
One-Step Synthesis Focus: Specifically designed for one-step synthesis, it provides concise and direct routes for your target compounds, streamlining the synthesis process.
Accurate Predictions: Utilizing the extensive PISTACHIO, BKMS_METABOLIC, PISTACHIO_RINGBREAKER, REAXYS, REAXYS_BIOCATALYSIS database, our tool offers high-accuracy predictions, reflecting the latest in chemical research and data.
Strategy Settings
Precursor scoring | Relevance Heuristic |
---|---|
Min. plausibility | 0.01 |
Model | Template_relevance |
Template Set | Pistachio/Bkms_metabolic/Pistachio_ringbreaker/Reaxys/Reaxys_biocatalysis |
Top-N result to add to graph | 6 |
Feasible Synthetic Routes
Q1: What is the biological significance of the Met-Asp sequence within cholecystokinin (CCK) peptides?
A1: The Met-Asp sequence is part of the crucial C-terminal tetrapeptide amide (Trp-Met-Asp-Phe-NH2) of CCK, which is essential for its biological activity. This tetrapeptide alone exhibits the full spectrum of CCK activity. [] This suggests that the Met-Asp sequence plays a vital role in receptor binding and activation.
Q2: How does the substitution of glycine for D-tryptophan or D-alanine at position 3 in CCK7 and CCK8 analogues affect their biological activity?
A2: These substitutions, particularly in Nα-carboxyacylated analogues, result in compounds that retain pancreatic exocrine secretion stimulation comparable to CCK8 while exhibiting significantly diminished gall bladder contracting activity. [] This suggests a dissociation of pancreozymin (PZ) activity from cholecystokinin (CCK) activity, highlighting the importance of the Met-Gly-Trp sequence for specific CCK functions.
Q3: Can organic acids replace the sulfate group in cholecystokinin (CCK-8) and still activate peripheral receptors?
A3: Research shows that replacing the sulfated tyrosine in CCK-8 with carboxy(alkyl)- or tetrazolyl(alkyl)-phenylalanine can maintain activity at the CCK-A receptor, albeit with lower potency compared to the natural substrate. [] This suggests that organic acids can fulfill the negative charge requirement at the receptor, opening avenues for designing novel CCK analogues.
Q4: What is the role of the Met-Asp bond in the context of amyloidogenic fragment production from the amyloid precursor protein (APP)?
A4: While lysosomal cathepsin B is known to degrade APP and produce amyloidogenic fragments, certain metalloendopeptidases might be involved in the turnover of intermediates. Specifically, phosphoramidon-insensitive metalloendopeptidases (PI-NEP) purified from rat brain show activity towards the Met-Asp bond in synthetic peptides mimicking the beta-secretase site. [] This suggests a potential role for Met-Asp cleavage in the complex amyloidogenic pathway.
Q5: Does the stereochemistry of the amino acid spacer preceding the Met-Asp sequence in minigastrin (MG) analogues influence their pharmacokinetics?
A5: Yes, studies using (111)In-labeled DOTA-conjugated MG analogues reveal that the stereochemistry of the N-terminal spacer significantly impacts their pharmacokinetics. The (d-Glu)6 spacer shows higher in vitro serum stability and an improved tumor-to-kidney ratio compared to the (l-Glu)6 counterpart, likely due to differences in secondary structure. [] These findings highlight the importance of stereochemistry in optimizing MG analogues for therapeutic purposes.
Q6: What is the minimal active sequence within cholecystokinin octapeptide (CCK8) required for reducing exploratory behavior?
A6: Research indicates that the entire C-terminal heptapeptide (Tyr(SO3H)-Met-Gly-Trp-Met-Asp-Phe-NH2) is necessary for the behavioral effects of CCK, contrasting with the activity of smaller fragments at other CCK receptors. [] This highlights the importance of the full heptapeptide sequence, including the Met-Asp motif, for specific CCK functions within the brain.
Q7: Does the presence of a sulfate group influence the conformational behavior of CCK peptides?
A8: Yes, sulfation of the tyrosine residue in CCK peptides, such as CCK7 and CCK8, enhances their tendency to adopt folded conformations, even in aqueous environments. [] This suggests that the sulfate group contributes to the stability of the biologically active conformation.
Q8: How does the conformation of the N-terminal tripeptide in dermenkephalin (DRE) relate to its delta-opioid receptor selectivity?
A9: Energy calculations and conformational analyses suggest that the C-terminal "delta-address" sequence (His-Leu-Met-Asp-NH2) in DRE influences the conformation of the N-terminal "mu-message" tripeptide. [] This interaction might explain the delta-opioid receptor selectivity of DRE despite sharing the N-terminal tripeptide with the mu-selective dermorphin.
Q9: What chromatographic techniques are employed for the purification and analysis of peptides containing the Met-Asp sequence?
A9: Various chromatographic techniques are employed, including:
- High-Performance Liquid Chromatography (HPLC): Widely used for purification and analysis, often with reverse-phase columns and specific solvent systems. [, , , ]
- Countercurrent Chromatography (CCC): Utilized for preparative-scale purification, particularly in the horizontal flow-through coil planet centrifuge format. []
- Gel Permeation Chromatography: Employed for initial separation based on molecular size, often using Sephadex G-50 columns. []
- Immunoaffinity Chromatography: Utilized for selective purification based on antibody-antigen interactions, often targeting specific CCK sequences. []
Q10: How does the use of 1,2-ethanedithiol (EDT) improve the solid-phase synthesis of peptides containing tryptophan?
A11: EDT facilitates the removal of the Nin-formyl protecting group from tryptophan during the liquid hydrogen fluoride (HF) cleavage step in solid-phase peptide synthesis. This method avoids the formation of side products and improves the yield of the desired peptide. [, ]
Disclaimer and Information on In-Vitro Research Products
Please be aware that all articles and product information presented on BenchChem are intended solely for informational purposes. The products available for purchase on BenchChem are specifically designed for in-vitro studies, which are conducted outside of living organisms. In-vitro studies, derived from the Latin term "in glass," involve experiments performed in controlled laboratory settings using cells or tissues. It is important to note that these products are not categorized as medicines or drugs, and they have not received approval from the FDA for the prevention, treatment, or cure of any medical condition, ailment, or disease. We must emphasize that any form of bodily introduction of these products into humans or animals is strictly prohibited by law. It is essential to adhere to these guidelines to ensure compliance with legal and ethical standards in research and experimentation.