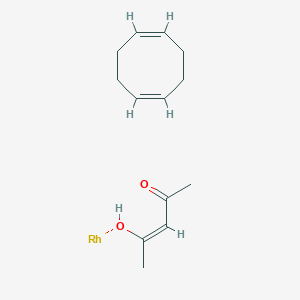
(Acetylacetonato)(1,5-cyclooctadiene)rhodium(I)
- Click on QUICK INQUIRY to receive a quote from our team of experts.
- With the quality product at a COMPETITIVE price, you can focus more on your research.
Overview
Description
(Acetylacetonato)(1,5-cyclooctadiene)rhodium(I) is a coordination compound that features rhodium in the +1 oxidation state. This compound is known for its catalytic properties and is widely used in various chemical reactions, particularly in organic synthesis and industrial applications. The compound has the molecular formula C13H19O2Rh and is characterized by its orange crystalline appearance .
Mechanism of Action
Target of Action
Rh(acac)(COD), also known as Acetylacetonato(1,5-cyclooctadiene)rhodium(I), is a coordination compound of rhodium. The primary targets of this compound are the molecules or atoms with which it interacts in chemical reactions .
Mode of Action
The compound interacts with its targets through a process known as oxidative addition . This process involves the addition of a molecule to the rhodium center, resulting in an increase in the oxidation state of the rhodium atom . The reaction of Rh(acac)(COD) with diimines and diphenylphosphine oxide leads to the formation of oxidative addition products .
Biochemical Pathways
The compound is known to participate in various chemical reactions, including those involving oxidative addition . These reactions can potentially influence a variety of biochemical pathways.
Pharmacokinetics
The compound’s molecular weight of 31019 suggests that it may have significant bioavailability.
Result of Action
The result of Rh(acac)(COD)'s action is the formation of new compounds through oxidative addition . For example, the reaction of Rh(acac)(COD) with diimines and diphenylphosphine oxide leads to the formation of hydrido-phosphinito-Rh(III) complexes .
Action Environment
The action of Rh(acac)(COD) can be influenced by various environmental factors. For instance, the compound’s reactivity can be affected by the presence of other molecules, the temperature, and the pH of the environment . Furthermore, Rh(acac)(COD) is used extensively in heterogeneous and homogeneous catalysis , suggesting that its action, efficacy, and stability can be significantly influenced by the specific conditions of the catalytic process.
Biochemical Analysis
Biochemical Properties
For example, rhodium complexes have been used as catalysts in biochemical reactions .
Cellular Effects
Other rhodium compounds have been shown to influence cell function, including impacts on cell signaling pathways, gene expression, and cellular metabolism .
Molecular Mechanism
Rhodium compounds are known to exert their effects at the molecular level, including binding interactions with biomolecules, enzyme inhibition or activation, and changes in gene expression .
Temporal Effects in Laboratory Settings
Other rhodium compounds have been shown to have long-term effects on cellular function in in vitro or in vivo studies .
Dosage Effects in Animal Models
Other rhodium compounds have been shown to have threshold effects and toxic or adverse effects at high doses .
Metabolic Pathways
Other rhodium compounds have been shown to interact with various enzymes and cofactors .
Transport and Distribution
Other rhodium compounds have been shown to interact with various transporters and binding proteins .
Subcellular Localization
Other rhodium compounds have been shown to be directed to specific compartments or organelles .
Preparation Methods
Synthetic Routes and Reaction Conditions
(Acetylacetonato)(1,5-cyclooctadiene)rhodium(I) can be synthesized through the reaction of rhodium trichloride with acetylacetone and 1,5-cyclooctadiene in the presence of a base. The reaction typically takes place in an inert atmosphere to prevent oxidation of the rhodium center. The product is then purified through recrystallization .
Industrial Production Methods
In industrial settings, the synthesis of (Acetylacetonato)(1,5-cyclooctadiene)rhodium(I) follows similar principles but is scaled up to accommodate larger quantities. The use of continuous flow reactors and automated systems ensures consistent quality and yield of the compound .
Chemical Reactions Analysis
Types of Reactions
(Acetylacetonato)(1,5-cyclooctadiene)rhodium(I) undergoes various types of chemical reactions, including:
Oxidation: The compound can be oxidized to higher oxidation states of rhodium.
Reduction: It can be reduced to form rhodium(0) complexes.
Substitution: Ligand substitution reactions are common, where the acetylacetonato or cyclooctadiene ligands are replaced by other ligands.
Common Reagents and Conditions
Oxidation: Common oxidizing agents include oxygen and hydrogen peroxide.
Reduction: Reducing agents such as hydrogen gas or sodium borohydride are used.
Substitution: Various ligands such as phosphines, amines, and carbonyls can be introduced under controlled conditions.
Major Products Formed
The major products formed from these reactions depend on the specific reagents and conditions used. For example, oxidation may yield rhodium(III) complexes, while substitution reactions can produce a wide range of rhodium(I) complexes with different ligands .
Scientific Research Applications
(Acetylacetonato)(1,5-cyclooctadiene)rhodium(I) is extensively used in scientific research due to its versatile catalytic properties. Some of its applications include:
Chemistry: It is used as a catalyst in hydrogenation, hydroformylation, and cross-coupling reactions.
Biology: The compound has been studied for its potential biological activity and interactions with biomolecules.
Medicine: Research is ongoing to explore its potential as an anticancer agent due to its ability to interact with DNA and proteins.
Industry: It is employed in the production of fine chemicals, pharmaceuticals, and polymers
Comparison with Similar Compounds
Similar Compounds
- (Acetylacetonato)dicarbonylrhodium(I)
- Rhodium(III) acetylacetonate
- Chloro(1,5-cyclooctadiene)rhodium(I) dimer
- Bis(acetonitrile)(1,5-cyclooctadiene)rhodium(I)tetrafluoroborate
Uniqueness
(Acetylacetonato)(1,5-cyclooctadiene)rhodium(I) is unique due to its combination of acetylacetonato and 1,5-cyclooctadiene ligands, which provide a balance of stability and reactivity. This makes it particularly effective as a catalyst in a wide range of chemical reactions, compared to other rhodium complexes that may have different ligand environments .
Properties
CAS No. |
12245-39-5 |
---|---|
Molecular Formula |
C13H20O2Rh |
Molecular Weight |
311.20 g/mol |
IUPAC Name |
cycloocta-1,5-diene;(Z)-4-hydroxypent-3-en-2-one;rhodium |
InChI |
InChI=1S/C8H12.C5H8O2.Rh/c1-2-4-6-8-7-5-3-1;1-4(6)3-5(2)7;/h1-2,7-8H,3-6H2;3,6H,1-2H3;/b;4-3-; |
InChI Key |
BUYVJWVYKPKZEX-LWFKIUJUSA-N |
Isomeric SMILES |
C/C(=C/C(=O)C)/O.C1CC=CCCC=C1.[Rh] |
SMILES |
CC(=CC(=O)C)O.C1CC=CCCC=C1.[Rh] |
Canonical SMILES |
CC(=CC(=O)C)O.C1CC=CCCC=C1.[Rh] |
Pictograms |
Irritant |
Synonyms |
acetylacetonate-1,5-cyclooctadiene rhodium I RhacacCod |
Origin of Product |
United States |
Retrosynthesis Analysis
AI-Powered Synthesis Planning: Our tool employs the Template_relevance Pistachio, Template_relevance Bkms_metabolic, Template_relevance Pistachio_ringbreaker, Template_relevance Reaxys, Template_relevance Reaxys_biocatalysis model, leveraging a vast database of chemical reactions to predict feasible synthetic routes.
One-Step Synthesis Focus: Specifically designed for one-step synthesis, it provides concise and direct routes for your target compounds, streamlining the synthesis process.
Accurate Predictions: Utilizing the extensive PISTACHIO, BKMS_METABOLIC, PISTACHIO_RINGBREAKER, REAXYS, REAXYS_BIOCATALYSIS database, our tool offers high-accuracy predictions, reflecting the latest in chemical research and data.
Strategy Settings
Precursor scoring | Relevance Heuristic |
---|---|
Min. plausibility | 0.01 |
Model | Template_relevance |
Template Set | Pistachio/Bkms_metabolic/Pistachio_ringbreaker/Reaxys/Reaxys_biocatalysis |
Top-N result to add to graph | 6 |
Feasible Synthetic Routes
Disclaimer and Information on In-Vitro Research Products
Please be aware that all articles and product information presented on BenchChem are intended solely for informational purposes. The products available for purchase on BenchChem are specifically designed for in-vitro studies, which are conducted outside of living organisms. In-vitro studies, derived from the Latin term "in glass," involve experiments performed in controlled laboratory settings using cells or tissues. It is important to note that these products are not categorized as medicines or drugs, and they have not received approval from the FDA for the prevention, treatment, or cure of any medical condition, ailment, or disease. We must emphasize that any form of bodily introduction of these products into humans or animals is strictly prohibited by law. It is essential to adhere to these guidelines to ensure compliance with legal and ethical standards in research and experimentation.