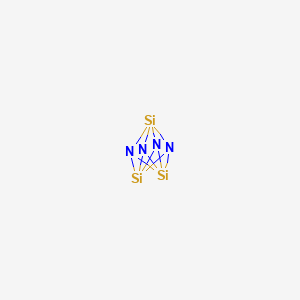
Silicon nitride
Overview
Description
Silicon nitride is a chemical compound composed of silicon and nitrogen. It is known for its high thermal stability, impressive mechanical strength, and resistance to wear and corrosion. This compound is typically found in a white, high-melting-point solid form and is relatively chemically inert. This compound is widely used in various industries due to its unique properties.
Mechanism of Action
Silicon nitride (Si3N4) is a chemical compound of the elements silicon and nitrogen . It is a white, high-melting-point solid that is relatively chemically inert . This article will explore the mechanism of action of this compound, covering its primary targets, mode of action, biochemical pathways, pharmacokinetics, results of action, and the influence of environmental factors.
Target of Action
This compound primarily targets a variety of applications due to its unique properties. It is used in clinics as an orthopedic implant material . In precise technologies of fabricating various types of electronic devices, this compound is utilized as thin films . It is also used for wear- and corrosion-resistant engine parts and accessory units, including turbochargers, glow plugs for diesel engines, exhaust gas control valves, and rocker arm pads for gas engines .
Mode of Action
This compound’s mode of action is primarily physical, leveraging its unique mechanical and chemical properties. It is prepared by heating powdered silicon between 1300 °C and 1400 °C in a nitrogen atmosphere . The silicon sample weight increases progressively due to the chemical combination of silicon and nitrogen . This compound and its hydrolysis products influence osteogenesis by modulating the biological behaviors of the constituents of the osteogenic microenvironment .
Biochemical Pathways
This compound regulates redox signaling, cellular autophagy, glycolysis, and bone mineralization in cells involved in bone formation via several mechanisms . Moreover, it may also promote osteogenesis by influencing immune regulation and angiogenesis .
Pharmacokinetics
As a bioceramic material, the molding process of this compound needs to be optimized . .
Result of Action
The osteogenesis-promoting properties of this compound are manifested in its contribution to the formation of a local osteogenic microenvironment . The surface of this compound can simultaneously inhibit the proliferation of bacteria while supporting the physiological activities of eukaryotic cells and promoting the healing of bone tissue .
Action Environment
The action of this compound is influenced by environmental factors such as temperature and pressure. For instance, this compound is prepared by heating powdered silicon between 1300 °C and 1400 °C in a nitrogen atmosphere . The wettability, surface morphology, and charge of this compound also play crucial roles in regulating its osteogenesis-promoting properties .
Biochemical Analysis
Biochemical Properties
Silicon nitride interacts with various biomolecules in biochemical reactions. It has been shown to exhibit excellent mechanical properties, cytocompatibility in vitro, and anti-bacterial properties
Cellular Effects
This compound has been shown to have no cytotoxicity and good cytocompatibility . It has been observed that there is good adhesion and cross-linking of cells during microwave-sintered this compound, and the morphology of the cytoskeleton was good .
Molecular Mechanism
It is known that this compound can interact with biomolecules and influence cellular functions .
Temporal Effects in Laboratory Settings
In laboratory settings, this compound has been shown to have stable effects over time. It has been prepared by a microwave sintering technique that was different from common production methods .
Preparation Methods
Synthetic Routes and Reaction Conditions: Silicon nitride can be synthesized through several methods, including direct nitridation, carbothermal reduction, and vapor-phase processes The most common method involves heating powdered silicon between 1300°C and 1400°C in a nitrogen atmosphere
Industrial Production Methods: In industrial settings, this compound is produced using low-pressure chemical vapor deposition (LPCVD) and plasma-enhanced chemical vapor deposition (PECVD). LPCVD operates at high temperatures, while PECVD works at lower temperatures and vacuum conditions. These methods are used to deposit this compound layers on semiconductor substrates .
Chemical Reactions Analysis
Types of Reactions: Silicon nitride undergoes various chemical reactions, including oxidation, reduction, and substitution. It is relatively inert but can be attacked by dilute hydrofluoric acid and hot phosphoric acid.
Common Reagents and Conditions:
Oxidation: this compound oxidizes at high temperatures, forming a protective silicon dioxide layer.
Reduction: Reduction reactions are less common but can occur under specific conditions.
Substitution: Substitution reactions involve replacing nitrogen atoms with other elements, often under high-temperature conditions.
Major Products: The primary product of this compound oxidation is silicon dioxide. Other reactions may produce various silicon and nitrogen compounds depending on the reagents and conditions used .
Scientific Research Applications
Silicon nitride has a wide range of applications in scientific research and industry:
Chemistry: Used as a catalyst support and in high-temperature reactions.
Biology: Biocompatible properties make it suitable for implants and surgical tools.
Medicine: Employed in orthopedic implants due to its strength and antibacterial properties.
Industry: Utilized in automotive and aerospace components, electronics, and cutting tools
Comparison with Similar Compounds
Silicon Carbide: Known for its hardness and thermal conductivity.
Silicon Dioxide: Commonly used in glass and semiconductor industries.
Boron Nitride: Exhibits excellent thermal and chemical stability
Silicon nitride’s unique combination of properties makes it a valuable material in various scientific and industrial applications. Its high thermal stability, mechanical strength, and biocompatibility set it apart from other similar compounds, making it a material of choice for many advanced applications.
Properties
IUPAC Name |
2,4,6,7-tetraza-1,3,5-trisilahexacyclo[3.1.1.01,4.02,5.03,6.03,7]heptane | |
---|---|---|
Source | PubChem | |
URL | https://pubchem.ncbi.nlm.nih.gov | |
Description | Data deposited in or computed by PubChem | |
InChI |
InChI=1S/N4Si3/c1-5-2-6(1)3(5)7(1,2)4(5)6 | |
Source | PubChem | |
URL | https://pubchem.ncbi.nlm.nih.gov | |
Description | Data deposited in or computed by PubChem | |
InChI Key |
HQVNEWCFYHHQES-UHFFFAOYSA-N | |
Source | PubChem | |
URL | https://pubchem.ncbi.nlm.nih.gov | |
Description | Data deposited in or computed by PubChem | |
Canonical SMILES |
N12[Si]34N5[Si]16N3[Si]25N46 | |
Source | PubChem | |
URL | https://pubchem.ncbi.nlm.nih.gov | |
Description | Data deposited in or computed by PubChem | |
Molecular Formula |
Si3N4, N4Si3 | |
Record name | silicon nitride | |
Source | Wikipedia | |
URL | https://en.wikipedia.org/wiki/Silicon_nitride | |
Description | Chemical information link to Wikipedia. | |
Source | PubChem | |
URL | https://pubchem.ncbi.nlm.nih.gov | |
Description | Data deposited in or computed by PubChem | |
DSSTOX Substance ID |
DTXSID20892247 | |
Record name | Silicon nitride (Si3N4) | |
Source | EPA DSSTox | |
URL | https://comptox.epa.gov/dashboard/DTXSID20892247 | |
Description | DSSTox provides a high quality public chemistry resource for supporting improved predictive toxicology. | |
Molecular Weight |
140.28 g/mol | |
Source | PubChem | |
URL | https://pubchem.ncbi.nlm.nih.gov | |
Description | Data deposited in or computed by PubChem | |
Physical Description |
White solid when pure, but may be brown or black; Exists in alpha and beta crystalline forms; [Merck Index] Grey odorless powder; [Alfa Aesar MSDS] | |
Record name | Silicon nitride | |
Source | Haz-Map, Information on Hazardous Chemicals and Occupational Diseases | |
URL | https://haz-map.com/Agents/18437 | |
Description | Haz-Map® is an occupational health database designed for health and safety professionals and for consumers seeking information about the adverse effects of workplace exposures to chemical and biological agents. | |
Explanation | Copyright (c) 2022 Haz-Map(R). All rights reserved. Unless otherwise indicated, all materials from Haz-Map are copyrighted by Haz-Map(R). No part of these materials, either text or image may be used for any purpose other than for personal use. Therefore, reproduction, modification, storage in a retrieval system or retransmission, in any form or by any means, electronic, mechanical or otherwise, for reasons other than personal use, is strictly prohibited without prior written permission. | |
CAS No. |
12033-89-5, 12033-60-2 | |
Record name | Silicon nitride | |
Source | ChemIDplus | |
URL | https://pubchem.ncbi.nlm.nih.gov/substance/?source=chemidplus&sourceid=0012033895 | |
Description | ChemIDplus is a free, web search system that provides access to the structure and nomenclature authority files used for the identification of chemical substances cited in National Library of Medicine (NLM) databases, including the TOXNET system. | |
Record name | Silicon nitride (Si3N4) | |
Source | EPA DSSTox | |
URL | https://comptox.epa.gov/dashboard/DTXSID20892247 | |
Description | DSSTox provides a high quality public chemistry resource for supporting improved predictive toxicology. | |
Record name | Silicon nitride | |
Source | European Chemicals Agency (ECHA) | |
URL | https://echa.europa.eu/substance-information/-/substanceinfo/100.031.612 | |
Description | The European Chemicals Agency (ECHA) is an agency of the European Union which is the driving force among regulatory authorities in implementing the EU's groundbreaking chemicals legislation for the benefit of human health and the environment as well as for innovation and competitiveness. | |
Explanation | Use of the information, documents and data from the ECHA website is subject to the terms and conditions of this Legal Notice, and subject to other binding limitations provided for under applicable law, the information, documents and data made available on the ECHA website may be reproduced, distributed and/or used, totally or in part, for non-commercial purposes provided that ECHA is acknowledged as the source: "Source: European Chemicals Agency, http://echa.europa.eu/". Such acknowledgement must be included in each copy of the material. ECHA permits and encourages organisations and individuals to create links to the ECHA website under the following cumulative conditions: Links can only be made to webpages that provide a link to the Legal Notice page. | |
Record name | SILICON NITRIDE | |
Source | FDA Global Substance Registration System (GSRS) | |
URL | https://gsrs.ncats.nih.gov/ginas/app/beta/substances/QHB8T06IDK | |
Description | The FDA Global Substance Registration System (GSRS) enables the efficient and accurate exchange of information on what substances are in regulated products. Instead of relying on names, which vary across regulatory domains, countries, and regions, the GSRS knowledge base makes it possible for substances to be defined by standardized, scientific descriptions. | |
Explanation | Unless otherwise noted, the contents of the FDA website (www.fda.gov), both text and graphics, are not copyrighted. They are in the public domain and may be republished, reprinted and otherwise used freely by anyone without the need to obtain permission from FDA. Credit to the U.S. Food and Drug Administration as the source is appreciated but not required. | |
Synthesis routes and methods I
Procedure details
Synthesis routes and methods II
Procedure details
Synthesis routes and methods III
Procedure details
Disclaimer and Information on In-Vitro Research Products
Please be aware that all articles and product information presented on BenchChem are intended solely for informational purposes. The products available for purchase on BenchChem are specifically designed for in-vitro studies, which are conducted outside of living organisms. In-vitro studies, derived from the Latin term "in glass," involve experiments performed in controlled laboratory settings using cells or tissues. It is important to note that these products are not categorized as medicines or drugs, and they have not received approval from the FDA for the prevention, treatment, or cure of any medical condition, ailment, or disease. We must emphasize that any form of bodily introduction of these products into humans or animals is strictly prohibited by law. It is essential to adhere to these guidelines to ensure compliance with legal and ethical standards in research and experimentation.