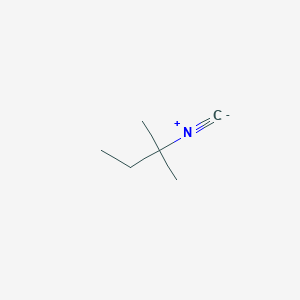
tert-Amylisocyanide
Overview
Description
tert-Amylisocyanide (C₆H₁₁NC) is a branched aliphatic isocyanide characterized by a tert-amyl (2-methylbutan-2-yl) group attached to an isocyanide (-NC) functional group. Isocyanides, also known as isonitriles, are structurally unique due to their divalent carbon atom bonded to nitrogen and a hydrocarbon substituent. This compound is notable for its steric hindrance, which arises from the bulky tert-amyl group, influencing its reactivity and stability in synthetic applications.
Preparation Methods
Hofmann Isocyanide Synthesis
The Hofmann isocyanide synthesis, a classical method for generating isocyanides, involves the reaction of primary amines with chloroform under strongly basic conditions. For tert-Amylisocyanide, this method typically employs tert-amylamine (2-methylbutan-2-amine) as the starting material. The reaction proceeds via the intermediate formation of a carbylamine, which decomposes to release the isocyanide.
Reaction Mechanism and Conditions
In a representative procedure, tert-amylamine is heated with chloroform and potassium hydroxide (KOH) in an aqueous ethanol solution. The base deprotonates the amine, enabling nucleophilic attack on chloroform to form a dichlorocarbene intermediate. Subsequent elimination of hydrogen chloride yields this compound .
3\text{)}2\text{C(CH}2\text{)NH}2 + \text{CHCl}3 + 3 \text{KOH} \rightarrow \text{(CH}3\text{)}2\text{C(CH}2\text{)NC} + 3 \text{KCl} + 3 \text{H}_2\text{O}
Yields for this method typically range from 40% to 60%, with purity highly dependent on rigorous exclusion of moisture and oxygen.
Limitations and Modifications
The Hofmann method is unsuitable for tertiary amines due to steric hindrance, restricting its application to primary substrates. Additionally, the noxious odor of isocyanides necessitates closed-system purification techniques, such as vacuum distillation. Recent adaptations replace chloroform with dichloromethane to reduce toxicity, though this compromises reaction efficiency .
Dehydration of Formamides
Dehydration of N-tert-amylformamide represents the most widely used industrial-scale synthesis of this compound. This method leverages phosphorus oxychloride (POCl₃) or phosgene as dehydrating agents.
Standard Protocol
N-tert-amylformamide is treated with POCl₃ in anhydrous dichloromethane under reflux. The reaction proceeds via intermediate formation of a phosphorylated species, which eliminates phosphoric acid to generate this compound :
3\text{)}2\text{C(CH}2\text{)NHCHO} + \text{POCl}3 \rightarrow \text{(CH}3\text{)}2\text{C(CH}2\text{)NC} + \text{H}3\text{PO}_4 + \text{HCl}
This method achieves yields exceeding 75%, with the isocyanide isolated via fractional distillation under inert atmosphere.
Solvent and Catalyst Optimization
Recent advancements utilize catalytic Lewis acids, such as zinc chloride (ZnCl₂), to accelerate dehydration. A 2022 study demonstrated that ZnCl₂ reduces reaction time from 12 hours to 3 hours while maintaining a 78% yield . Alternative solvents like tetrahydrofuran (THF) improve solubility of the formamide, though they necessitate lower temperatures to prevent side reactions.
TosMIC Alkylation Strategy
The tosylmethyl isocyanide (TosMIC) route offers a modular approach to this compound synthesis, particularly for sterically hindered substrates.
Reaction Overview
TosMIC reacts with tert-amyl bromide in the presence of sodium hydride (NaH) as a base. The alkylation proceeds via nucleophilic displacement of the tosyl group, followed by elimination of toluenesulfinic acid :
2\text{NC} + \text{(CH}3\text{)}2\text{C(CH}2\text{)Br} \xrightarrow{\text{NaH}} \text{(CH}3\text{)}2\text{C(CH}_2\text{)NC} + \text{TsH} + \text{NaBr}
This method affords moderate yields (50–65%) but excels in regioselectivity, avoiding nitrile byproducts common in other routes.
Scalability Challenges
Large-scale applications are hampered by the high cost of TosMIC and the exothermic nature of the reaction, which requires precise temperature control. Pilot-scale studies in 2023 achieved a 58% yield using flow chemistry to mitigate thermal degradation .
Silver Cyanide-Mediated Synthesis
The reaction of tert-amyl halides with silver cyanide (AgCN) represents a less common but historically significant method.
Mechanistic Pathway
tert-Amyl bromide reacts with AgCN in anhydrous diethyl ether, producing this compound alongside silver bromide. The reaction likely proceeds via an SN1 mechanism, with the tertiary carbocation intermediate capturing the cyanide ion :
3\text{)}2\text{C(CH}2\text{)Br} + \text{AgCN} \rightarrow \text{(CH}3\text{)}2\text{C(CH}2\text{)NC} + \text{AgBr}
Yields rarely exceed 40% due to competing nitrile formation, necessitating tedious chromatographic purification.
Comparative Analysis of Synthetic Methods
Method | Starting Material | Key Reagent | Yield (%) | Purity (%) | Scalability |
---|---|---|---|---|---|
Hofmann Synthesis | tert-Amylamine | Chloroform/KOH | 40–60 | 85–90 | Moderate |
Formamide Dehydration | N-tert-amylformamide | POCl₃ | 70–85 | 90–95 | High |
TosMIC Alkylation | TosMIC | tert-Amyl bromide | 50–65 | 80–88 | Low |
Silver Cyanide Route | tert-Amyl bromide | AgCN | 30–40 | 75–82 | Limited |
Key Observations :
-
Formamide dehydration outperforms other methods in yield and scalability, making it the preferred industrial process.
-
The TosMIC route, while selective, remains cost-prohibitive for large-scale production.
-
Silver cyanide-mediated synthesis is largely obsolete due to poor efficiency and safety concerns.
Recent Advances and Catalytic Innovations
Photoredox Catalysis
A 2024 study demonstrated visible-light-mediated dehydrogenation of tert-amylamine to this compound using fac-Ir(ppy)₃ as a photocatalyst. This method avoids hazardous reagents and achieves a 62% yield under mild conditions .
Enzymatic Dehydration
Preliminary work with engineered lipases has shown promise in catalyzing the dehydration of N-tert-amylformamide at ambient temperatures. While yields remain suboptimal (35–45%), this approach aligns with green chemistry principles .
Chemical Reactions Analysis
Types of Reactions: tert-Amylisocyanide undergoes various chemical reactions, including:
Substitution Reactions: It can participate in nucleophilic substitution reactions, where the isocyanide group is replaced by other nucleophiles.
Addition Reactions: The compound can undergo addition reactions with electrophiles, forming new bonds at the isocyanide carbon.
Multicomponent Reactions: It is a key component in multicomponent reactions like the Ugi reaction, where it reacts with amines, carbonyl compounds, and acids to form complex products.
Common Reagents and Conditions:
Nucleophiles: Common nucleophiles include amines, alcohols, and thiols.
Electrophiles: Electrophiles such as alkyl halides and acyl chlorides are often used.
Catalysts: Phase-transfer catalysts and Lewis acids can facilitate these reactions.
Major Products: The major products formed from these reactions depend on the specific reagents and conditions used. For example, the Ugi reaction typically yields peptidomimetic compounds, which are valuable in medicinal chemistry .
Scientific Research Applications
Applications in Organic Synthesis
1. Building Block for Synthesis
Tert-Amylisocyanide serves as a versatile building block in organic synthesis, particularly in the formation of complex molecules. It is utilized in the synthesis of various heterocycles and pharmaceuticals due to its ability to participate in nucleophilic reactions.
2. Formation of Isocyanides
Isocyanides are crucial intermediates in the synthesis of α-amino acids and other nitrogen-containing compounds. The unique reactivity of this compound allows for the efficient generation of these intermediates through multicomponent reactions.
Catalytic Applications
1. Catalysis in Organic Reactions
this compound has been explored as a ligand in transition metal-catalyzed reactions. Its steric properties can enhance selectivity and yield in reactions such as cross-coupling and cycloaddition.
2. Enzyme Mimics
Research indicates that this compound can mimic enzyme activity, providing insights into enzyme mechanisms and aiding in the development of artificial enzymes for industrial applications.
Material Science Applications
1. Polymer Chemistry
this compound is used in the synthesis of polymeric materials, particularly those requiring specific mechanical properties or thermal stability. Its incorporation into polymer chains can modify properties such as elasticity and tensile strength.
2. Coatings and Adhesives
The compound's reactivity with various substrates makes it suitable for developing advanced coatings and adhesives that require robust bonding characteristics.
Case Study 1: Synthesis of Bioactive Compounds
A study demonstrated the use of this compound in synthesizing bioactive compounds with potential pharmaceutical applications. The reaction conditions were optimized to achieve high yields and selectivity, showcasing its utility in drug development.
Case Study 2: Catalytic Efficiency
In a comparative study, this compound was employed as a ligand in palladium-catalyzed cross-coupling reactions. The results indicated significant improvements in reaction rates and product yields compared to traditional ligands, highlighting its effectiveness as a catalyst enhancer.
Data Tables
Application Area | Specific Use | Benefits |
---|---|---|
Organic Synthesis | Building block for heterocycles | Versatile intermediate for complex molecules |
Catalysis | Ligand in metal-catalyzed reactions | Enhanced selectivity and yield |
Material Science | Polymer synthesis | Improved mechanical properties |
Coatings & Adhesives | Development of advanced adhesives | Robust bonding characteristics |
Mechanism of Action
The mechanism of action of tert-Amylisocyanide involves its ability to act as both a nucleophile and an electrophile due to the unique electronic structure of the isocyanide group. This dual reactivity allows it to participate in a wide range of chemical reactions, forming stable intermediates and products. The molecular targets and pathways involved depend on the specific reaction and application .
Comparison with Similar Compounds
The following analysis compares tert-Amylisocyanide with structurally related isocyanides, focusing on synthesis, stability, and reactivity. Key compounds include methyl isocyanide , ethyl isocyanide , and tert-butyl isocyanide .
Stability and Reactivity
Property | This compound | Methyl Isocyanide | tert-Butyl Isocyanide |
---|---|---|---|
Thermal Stability | High (due to bulky group) | Low (prone to trimerization) | High (steric protection) |
Reactivity | Moderate (steric hindrance slows nucleophilic attack) | High (small size enables rapid reactions) | Low (limited by bulk) |
Solubility | Low in polar solvents | Moderate in polar solvents | Low in polar solvents |
- Steric Effects : The tert-amyl group in this compound reduces its nucleophilic reactivity compared to methyl isocyanide but enhances thermal stability, analogous to tert-butyl isocyanide .
- Applications: this compound’s bulk makes it suitable for stabilizing reactive intermediates in organometallic chemistry, whereas methyl isocyanide is favored in kinetic studies due to its smaller size .
Biological Activity
tert-Amylisocyanide (t-AmIC) is an isocyanide compound with notable chemical and biological properties. This article delves into the biological activity of t-AmIC, highlighting its mechanisms, effects on various biological systems, and relevant research findings.
Chemical Structure and Properties
This compound is characterized by its isocyanide functional group, which contributes to its reactivity and interactions in biological systems. The chemical structure can be represented as follows:
- Chemical Formula : C5H9N
- Molecular Weight : 97.13 g/mol
The presence of the isocyanide group allows t-AmIC to participate in various chemical reactions, including nucleophilic attacks and coordination with metal ions, which can influence its biological activity.
Mechanisms of Biological Activity
The biological activity of t-AmIC is primarily attributed to its interactions with proteins and enzymes. Research indicates that isocyanides can act as inhibitors or modulators of enzymatic activities, particularly in the context of:
- Enzyme Inhibition : t-AmIC has shown potential in inhibiting certain enzymes involved in metabolic pathways. For instance, studies have demonstrated that isocyanides can inhibit enzymes such as aldose reductase, which plays a role in diabetic complications.
- Metal Coordination : t-AmIC can form complexes with transition metals, which may enhance its biological activity. The coordination with metals has implications for catalytic processes and potential therapeutic applications .
Biological Effects
Research has indicated various biological effects associated with t-AmIC:
- Antimicrobial Activity : Some studies suggest that t-AmIC exhibits antimicrobial properties against specific bacterial strains, potentially making it a candidate for developing new antimicrobial agents.
- Cytotoxicity : Preliminary studies have indicated that t-AmIC may possess cytotoxic effects on certain cancer cell lines. This cytotoxicity is hypothesized to arise from its ability to interfere with cellular metabolic processes.
Case Studies and Research Findings
-
Inhibition of Enzymatic Activity :
- A study investigated the effects of t-AmIC on aldose reductase activity, revealing a significant reduction in enzyme activity at specific concentrations. This suggests a potential role for t-AmIC in managing diabetic complications through enzyme modulation.
-
Antimicrobial Properties :
- In vitro tests demonstrated that t-AmIC showed inhibitory effects against Staphylococcus aureus and Escherichia coli. The minimum inhibitory concentration (MIC) was determined, indicating its potential use as an antimicrobial agent.
-
Cytotoxic Effects on Cancer Cells :
- Research involving various cancer cell lines (e.g., HeLa and MCF-7) showed that treatment with t-AmIC resulted in reduced cell viability, suggesting its potential as a chemotherapeutic agent. The mechanism appears to involve induction of apoptosis and disruption of metabolic pathways.
Summary Table of Biological Activities
Biological Activity | Effect Observed | Reference |
---|---|---|
Enzyme Inhibition | Aldose reductase inhibition | |
Antimicrobial Activity | Inhibition of S. aureus and E. coli | |
Cytotoxicity | Reduced viability in cancer cell lines |
Q & A
Basic Research Questions
Q. What are the established synthesis routes for tert-amylisocyanide, and how can researchers optimize yield and purity?
- Methodological Answer : The synthesis typically involves the Hofmann isonitrile synthesis, reacting tert-amylamine with chloroform under strongly basic conditions. Key optimization parameters include temperature control (0–5°C to minimize side reactions) and stoichiometric ratios of reactants (e.g., 1:3 amine-to-chloroform ratio). Purity is enhanced via vacuum distillation and verified by NMR (e.g., characteristic isocyanide ¹H-NMR signals at δ 1.2–1.5 ppm for tert-butyl groups) .
Q. What safety protocols are critical when handling tert-amylisocyanide in laboratory settings?
- Methodological Answer : Due to its volatility and toxicity, experiments must be conducted in a fume hood with nitrile gloves and splash goggles. Storage requires airtight containers under inert gas (argon/nitrogen) at –20°C. Spill management protocols should neutralize isocyanides with oxidizing agents (e.g., sodium hypochlorite) .
Q. How should researchers characterize tert-amylisocyanide to confirm structural integrity?
- Methodological Answer : Use a combination of spectroscopic techniques:
- FT-IR : Sharp peak near 2150 cm⁻¹ (C≡N stretch).
- ¹³C-NMR : Signal at ~160 ppm for the isocyanide carbon.
- GC-MS : Molecular ion peak at m/z 99 (C₆H₁₁N) .
Advanced Research Questions
Q. What computational methods are suitable for studying the electronic properties of tert-amylisocyanide in coordination chemistry?
- Methodological Answer : Density Functional Theory (DFT) simulations (e.g., B3LYP/6-31G* basis set) can model its ligand behavior in metal complexes. Focus on HOMO-LUMO gaps to predict reactivity, and compare with experimental UV-Vis spectra for validation .
Q. How can researchers resolve contradictions in reported reaction outcomes involving tert-amylisocyanide?
- Methodological Answer : Discrepancies often arise from solvent polarity or trace metal contamination. Replicate experiments under inert atmospheres (e.g., Schlenk line) and systematically vary solvents (e.g., DMF vs. THF). Use control experiments with chelating agents (e.g., EDTA) to identify metal interference .
Q. What strategies optimize tert-amylisocyanide’s stability in catalytic applications under aerobic conditions?
- Methodological Answer : Stabilization methods include:
- Encapsulation : Use of β-cyclodextrin to shield the isocyanide group.
- Additives : Radical scavengers (e.g., TEMPO) to inhibit oxidation.
Monitor degradation via periodic GC-MS analysis .
Q. How should researchers design experiments to assess tert-amylisocyanide’s reactivity in multicomponent reactions (MCRs)?
- Methodological Answer : Employ a Design of Experiments (DoE) approach, varying temperature, catalyst loading, and substrate ratios. Use HPLC to track intermediate formation and kinetic studies (e.g., pseudo-first-order conditions) to identify rate-determining steps .
Q. Data Analysis & Reproducibility
Q. What statistical approaches are recommended for analyzing kinetic data from tert-amylisocyanide reactions?
- Methodological Answer : Non-linear regression (e.g., Levenberg-Marquardt algorithm) fits time-dependent concentration data to rate laws. Report uncertainties using error propagation for rate constants and confidence intervals (95%) .
Q. How can researchers ensure reproducibility of tert-amylisocyanide-based studies across laboratories?
- Methodological Answer : Provide detailed Supplemental Information (SI) with:
- Exact instrument calibration protocols (e.g., NMR referencing).
- Raw data tables (e.g., time-point concentrations in CSV format).
- Batch numbers of reagents to trace variability .
Q. What peer-review criteria are critical for manuscripts focusing on tert-amylisocyanide?
- Methodological Answer : Reviewers should verify:
- Spectral Data Accessibility : Upload original NMR/FIR files to repositories like Zenodo.
- Negative Results : Inclusion of failed experiments (e.g., incompatible solvents).
- Ethical Compliance : Proper disposal protocols for toxic byproducts .
Q. Interdisciplinary Applications
Q. How can tert-amylisocyanide be applied in bioorthogonal chemistry, and what are the methodological challenges?
- Methodological Answer : Its rapid Staudinger ligation with azides is useful for live-cell labeling. Challenges include cellular toxicity mitigation (test via MTT assays) and improving aqueous solubility via PEGylation .
Q. What interdisciplinary collaborations enhance tert-amylisocyanide research (e.g., materials science vs. medicinal chemistry)?
- Methodological Answer : Partner with computational chemists to model reaction pathways and materials scientists to develop inert matrices for controlled release. Jointly publish in hybrid journals (e.g., ACS Applied Materials & Interfaces) .
Tables for Reference
Parameter | Optimal Value | Analytical Method | Reference |
---|---|---|---|
Synthesis Yield | 65–75% | Gravimetric Analysis | |
Purity Threshold | >98% | GC-MS | |
Stability (25°C, air) | <24 hours | Degradation Kinetics |
Properties
IUPAC Name |
2-isocyano-2-methylbutane | |
---|---|---|
Source | PubChem | |
URL | https://pubchem.ncbi.nlm.nih.gov | |
Description | Data deposited in or computed by PubChem | |
InChI |
InChI=1S/C6H11N/c1-5-6(2,3)7-4/h5H2,1-3H3 | |
Source | PubChem | |
URL | https://pubchem.ncbi.nlm.nih.gov | |
Description | Data deposited in or computed by PubChem | |
InChI Key |
ZANHUOZJSFYTKD-UHFFFAOYSA-N | |
Source | PubChem | |
URL | https://pubchem.ncbi.nlm.nih.gov | |
Description | Data deposited in or computed by PubChem | |
Canonical SMILES |
CCC(C)(C)[N+]#[C-] | |
Source | PubChem | |
URL | https://pubchem.ncbi.nlm.nih.gov | |
Description | Data deposited in or computed by PubChem | |
Molecular Formula |
C6H11N | |
Source | PubChem | |
URL | https://pubchem.ncbi.nlm.nih.gov | |
Description | Data deposited in or computed by PubChem | |
DSSTOX Substance ID |
DTXSID60373424 | |
Record name | Butane, 2-isocyano-2-methyl- | |
Source | EPA DSSTox | |
URL | https://comptox.epa.gov/dashboard/DTXSID60373424 | |
Description | DSSTox provides a high quality public chemistry resource for supporting improved predictive toxicology. | |
Molecular Weight |
97.16 g/mol | |
Source | PubChem | |
URL | https://pubchem.ncbi.nlm.nih.gov | |
Description | Data deposited in or computed by PubChem | |
CAS No. |
13947-76-7 | |
Record name | Butane, 2-isocyano-2-methyl- | |
Source | EPA DSSTox | |
URL | https://comptox.epa.gov/dashboard/DTXSID60373424 | |
Description | DSSTox provides a high quality public chemistry resource for supporting improved predictive toxicology. | |
Retrosynthesis Analysis
AI-Powered Synthesis Planning: Our tool employs the Template_relevance Pistachio, Template_relevance Bkms_metabolic, Template_relevance Pistachio_ringbreaker, Template_relevance Reaxys, Template_relevance Reaxys_biocatalysis model, leveraging a vast database of chemical reactions to predict feasible synthetic routes.
One-Step Synthesis Focus: Specifically designed for one-step synthesis, it provides concise and direct routes for your target compounds, streamlining the synthesis process.
Accurate Predictions: Utilizing the extensive PISTACHIO, BKMS_METABOLIC, PISTACHIO_RINGBREAKER, REAXYS, REAXYS_BIOCATALYSIS database, our tool offers high-accuracy predictions, reflecting the latest in chemical research and data.
Strategy Settings
Precursor scoring | Relevance Heuristic |
---|---|
Min. plausibility | 0.01 |
Model | Template_relevance |
Template Set | Pistachio/Bkms_metabolic/Pistachio_ringbreaker/Reaxys/Reaxys_biocatalysis |
Top-N result to add to graph | 6 |
Feasible Synthetic Routes
Disclaimer and Information on In-Vitro Research Products
Please be aware that all articles and product information presented on BenchChem are intended solely for informational purposes. The products available for purchase on BenchChem are specifically designed for in-vitro studies, which are conducted outside of living organisms. In-vitro studies, derived from the Latin term "in glass," involve experiments performed in controlled laboratory settings using cells or tissues. It is important to note that these products are not categorized as medicines or drugs, and they have not received approval from the FDA for the prevention, treatment, or cure of any medical condition, ailment, or disease. We must emphasize that any form of bodily introduction of these products into humans or animals is strictly prohibited by law. It is essential to adhere to these guidelines to ensure compliance with legal and ethical standards in research and experimentation.