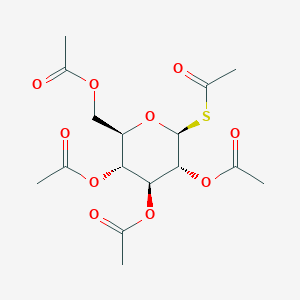
1-Thio-beta-D-glucose pentaacetate
Overview
Description
1-Thio-beta-D-glucose pentaacetate is a derivative of glucose, where the hydroxyl group at the anomeric carbon is replaced by a thio group (sulfur atom) and all hydroxyl groups are acetylated.
Mechanism of Action
Target of Action
The primary target of 1-Thio-beta-D-glucose pentaacetate is glucose oxidase (GOx), an enzyme that catalyzes the oxidation of glucose . This interaction plays a crucial role in the compound’s mechanism of action.
Mode of Action
This compound interacts with its target, GOx, by wrapping around it . This interaction leads to the generation of cytotoxic hydrogen sulfide (H2S) and hydrogen peroxide (H2O2), which are activated by glutathione . The resulting changes include the suppression of cytochrome c oxidase expression and damage to the mitochondrial membrane potential .
Biochemical Pathways
The biochemical pathway affected by this compound involves the glycolysis process. The compound, through its interaction with GOx, blocks glycolysis by depleting endogenous glucose . This action leads to a complete depletion of tumorigenic energy sources .
Result of Action
The result of this compound’s action is the induction of synergistic defects in mitochondrial function . This is achieved through the suppression of cytochrome c oxidase expression and damage to the mitochondrial membrane potential . These molecular and cellular effects contribute to the compound’s overall action.
Biochemical Analysis
Biochemical Properties
It is known that the sulfur atom in the molecule can potentially interact with various enzymes, proteins, and other biomolecules . The nature of these interactions is likely to be influenced by the unique chemical properties of sulfur, such as its ability to form strong covalent bonds.
Cellular Effects
Given its structural similarity to glucose, it may influence cell function by interacting with glucose transporters and enzymes involved in glucose metabolism
Molecular Mechanism
It is possible that the compound exerts its effects at the molecular level through binding interactions with biomolecules, enzyme inhibition or activation, and changes in gene expression
Temporal Effects in Laboratory Settings
Future in vitro and in vivo studies could provide valuable insights into these aspects .
Dosage Effects in Animal Models
There is currently limited information available on the effects of different dosages of 1-Thio-beta-D-glucose Pentaacetate in animal models. Future studies could investigate potential threshold effects, as well as any toxic or adverse effects at high doses .
Metabolic Pathways
The metabolic pathways involving this compound are not well characterized. Given its structural similarity to glucose, it may be involved in similar metabolic pathways, potentially interacting with enzymes or cofactors involved in glucose metabolism .
Transport and Distribution
It is possible that it interacts with glucose transporters and is distributed similarly to glucose within cells .
Subcellular Localization
The subcellular localization of this compound is not well known. Future studies could investigate whether it is directed to specific compartments or organelles within the cell .
Preparation Methods
Synthetic Routes and Reaction Conditions: 1-Thio-beta-D-glucose pentaacetate can be synthesized through a multi-step process starting from D-glucose. The synthesis involves the protection of hydroxyl groups by acetylation, followed by the introduction of the thio group. A typical synthetic route includes:
Acetylation of D-glucose: D-glucose is reacted with acetic anhydride in the presence of a catalyst like pyridine to form glucose pentaacetate.
Introduction of Thio Group: The glucose pentaacetate is then treated with a thio reagent, such as thioacetic acid, under specific conditions to replace the anomeric hydroxyl group with a thio group, resulting in this compound
Industrial Production Methods: While specific industrial production methods for this compound are not widely documented, the general approach involves scaling up the laboratory synthesis procedures. This includes optimizing reaction conditions, using industrial-grade reagents, and employing large-scale reactors to ensure high yield and purity .
Chemical Reactions Analysis
1-Thio-beta-D-glucose pentaacetate undergoes various chemical reactions, including:
Oxidation: The thio group can be oxidized to form sulfoxides or sulfones using oxidizing agents like hydrogen peroxide or m-chloroperbenzoic acid.
Reduction: The compound can be reduced to form the corresponding thiol using reducing agents such as lithium aluminum hydride.
Substitution: The acetyl groups can be substituted with other functional groups through nucleophilic substitution reactions. .
Major Products Formed:
Sulfoxides and Sulfones: Formed through oxidation reactions.
Thiols: Formed through reduction reactions.
Deacetylated Derivatives: Formed through substitution reactions
Scientific Research Applications
1-Thio-beta-D-glucose pentaacetate has several scientific research applications:
Chemistry: Used as a building block in the synthesis of complex carbohydrates and glycosides. .
Biology: Employed in the study of glycosidase inhibitors and activators.
Industry: Used in the synthesis of specialty chemicals and as an intermediate in the production of other bioactive compounds
Comparison with Similar Compounds
1-Thio-beta-D-glucose pentaacetate can be compared with other similar compounds, such as:
1-Thio-beta-D-glucose tetraacetate: Similar structure but with one less acetyl group.
Beta-D-glucose pentaacetate: Lacks the thio group, making it less reactive in certain chemical reactions.
Alpha-D-glucose pentaacetate: Different anomeric configuration, leading to different stereochemistry and reactivity.
Uniqueness: this compound is unique due to the presence of the thio group, which imparts distinct chemical properties and reactivity. This makes it valuable in specific synthetic and research applications where other glucose derivatives may not be suitable .
Properties
IUPAC Name |
(3,4,5-triacetyloxy-6-acetylsulfanyloxan-2-yl)methyl acetate | |
---|---|---|
Details | Computed by Lexichem TK 2.7.0 (PubChem release 2021.05.07) | |
Source | PubChem | |
URL | https://pubchem.ncbi.nlm.nih.gov | |
Description | Data deposited in or computed by PubChem | |
InChI |
InChI=1S/C16H22O10S/c1-7(17)22-6-12-13(23-8(2)18)14(24-9(3)19)15(25-10(4)20)16(26-12)27-11(5)21/h12-16H,6H2,1-5H3 | |
Details | Computed by InChI 1.0.6 (PubChem release 2021.05.07) | |
Source | PubChem | |
URL | https://pubchem.ncbi.nlm.nih.gov | |
Description | Data deposited in or computed by PubChem | |
InChI Key |
CFAJEDWNNGFOQV-UHFFFAOYSA-N | |
Details | Computed by InChI 1.0.6 (PubChem release 2021.05.07) | |
Source | PubChem | |
URL | https://pubchem.ncbi.nlm.nih.gov | |
Description | Data deposited in or computed by PubChem | |
Canonical SMILES |
CC(=O)OCC1C(C(C(C(O1)SC(=O)C)OC(=O)C)OC(=O)C)OC(=O)C | |
Details | Computed by OEChem 2.3.0 (PubChem release 2021.05.07) | |
Source | PubChem | |
URL | https://pubchem.ncbi.nlm.nih.gov | |
Description | Data deposited in or computed by PubChem | |
Molecular Formula |
C16H22O10S | |
Details | Computed by PubChem 2.1 (PubChem release 2021.05.07) | |
Source | PubChem | |
URL | https://pubchem.ncbi.nlm.nih.gov | |
Description | Data deposited in or computed by PubChem | |
DSSTOX Substance ID |
DTXSID70929370 | |
Record name | 2,3,4,6-Tetra-O-acetyl-1-S-acetyl-1-thiohexopyranose | |
Source | EPA DSSTox | |
URL | https://comptox.epa.gov/dashboard/DTXSID70929370 | |
Description | DSSTox provides a high quality public chemistry resource for supporting improved predictive toxicology. | |
Molecular Weight |
406.4 g/mol | |
Details | Computed by PubChem 2.1 (PubChem release 2021.05.07) | |
Source | PubChem | |
URL | https://pubchem.ncbi.nlm.nih.gov | |
Description | Data deposited in or computed by PubChem | |
CAS No. |
13639-50-4, 6806-56-0 | |
Record name | NSC409737 | |
Source | DTP/NCI | |
URL | https://dtp.cancer.gov/dtpstandard/servlet/dwindex?searchtype=NSC&outputformat=html&searchlist=409737 | |
Description | The NCI Development Therapeutics Program (DTP) provides services and resources to the academic and private-sector research communities worldwide to facilitate the discovery and development of new cancer therapeutic agents. | |
Explanation | Unless otherwise indicated, all text within NCI products is free of copyright and may be reused without our permission. Credit the National Cancer Institute as the source. | |
Record name | NSC89877 | |
Source | DTP/NCI | |
URL | https://dtp.cancer.gov/dtpstandard/servlet/dwindex?searchtype=NSC&outputformat=html&searchlist=89877 | |
Description | The NCI Development Therapeutics Program (DTP) provides services and resources to the academic and private-sector research communities worldwide to facilitate the discovery and development of new cancer therapeutic agents. | |
Explanation | Unless otherwise indicated, all text within NCI products is free of copyright and may be reused without our permission. Credit the National Cancer Institute as the source. | |
Record name | 2,3,4,6-Tetra-O-acetyl-1-S-acetyl-1-thiohexopyranose | |
Source | EPA DSSTox | |
URL | https://comptox.epa.gov/dashboard/DTXSID70929370 | |
Description | DSSTox provides a high quality public chemistry resource for supporting improved predictive toxicology. | |
Disclaimer and Information on In-Vitro Research Products
Please be aware that all articles and product information presented on BenchChem are intended solely for informational purposes. The products available for purchase on BenchChem are specifically designed for in-vitro studies, which are conducted outside of living organisms. In-vitro studies, derived from the Latin term "in glass," involve experiments performed in controlled laboratory settings using cells or tissues. It is important to note that these products are not categorized as medicines or drugs, and they have not received approval from the FDA for the prevention, treatment, or cure of any medical condition, ailment, or disease. We must emphasize that any form of bodily introduction of these products into humans or animals is strictly prohibited by law. It is essential to adhere to these guidelines to ensure compliance with legal and ethical standards in research and experimentation.