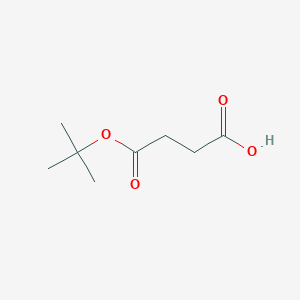
mono-tert-Butyl succinate
Overview
Description
Mono-tert-Butyl succinate: is an organic compound with the molecular formula C8H14O4. It is a derivative of succinic acid, where one of the carboxyl groups is esterified with tert-butyl alcohol. This compound is used as an intermediate in organic synthesis and has applications in various fields, including chemistry and biology.
Preparation Methods
Synthetic Routes and Reaction Conditions: Mono-tert-Butyl succinate can be synthesized by refluxing a mixture of succinic anhydride and N-hydroxyl succinimide in the presence of dimethylaminopyridine and triethylamine in tert-butanol and toluene . The reaction typically involves heating the mixture to facilitate the esterification process.
Industrial Production Methods: In industrial settings, the production of this compound may involve similar esterification reactions but on a larger scale. The use of continuous flow reactors and optimized reaction conditions can enhance yield and purity. The process may also involve purification steps such as distillation or crystallization to obtain the desired product.
Chemical Reactions Analysis
Types of Reactions: Mono-tert-Butyl succinate undergoes various chemical reactions, including:
Hydrolysis: The ester group can be hydrolyzed to yield succinic acid and tert-butyl alcohol.
Oxidation: The compound can be oxidized to form different oxidation products depending on the reagents and conditions used.
Substitution: The tert-butyl group can be substituted with other functional groups under appropriate conditions.
Common Reagents and Conditions:
Hydrolysis: Acidic or basic conditions can be used for hydrolysis. Common reagents include hydrochloric acid or sodium hydroxide.
Oxidation: Oxidizing agents such as potassium permanganate or chromium trioxide can be used.
Substitution: Various nucleophiles can be used for substitution reactions, depending on the desired product.
Major Products Formed:
Hydrolysis: Succinic acid and tert-butyl alcohol.
Oxidation: Depending on the conditions, products may include succinic acid derivatives or other oxidized forms.
Substitution: Products vary based on the nucleophile used in the reaction.
Scientific Research Applications
Mono-tert-Butyl succinate has several applications in scientific research:
Chemistry: It is used as an intermediate in the synthesis of various organic compounds, including pharmaceuticals and agrochemicals.
Biology: The compound is used in the preparation of peptides and other biologically active molecules.
Medicine: It serves as a building block for the synthesis of drug candidates and other therapeutic agents.
Industry: this compound is used in the production of polymers, resins, and other industrial materials.
Mechanism of Action
The mechanism of action of mono-tert-Butyl succinate depends on its specific application. In organic synthesis, it acts as an intermediate, participating in various chemical reactions to form desired products. The molecular targets and pathways involved vary based on the specific reactions and conditions used.
Comparison with Similar Compounds
Mono-ethyl succinate: Similar to mono-tert-Butyl succinate but with an ethyl group instead of a tert-butyl group.
Di-tert-butyl succinate: Contains two tert-butyl ester groups.
Mono-tert-butyl malonate: Similar structure but derived from malonic acid instead of succinic acid.
Uniqueness: this compound is unique due to its specific esterification with tert-butyl alcohol, which imparts distinct chemical properties and reactivity. Its applications in organic synthesis and industrial processes highlight its versatility and importance in various fields .
Properties
IUPAC Name |
4-[(2-methylpropan-2-yl)oxy]-4-oxobutanoic acid | |
---|---|---|
Source | PubChem | |
URL | https://pubchem.ncbi.nlm.nih.gov | |
Description | Data deposited in or computed by PubChem | |
InChI |
InChI=1S/C8H14O4/c1-8(2,3)12-7(11)5-4-6(9)10/h4-5H2,1-3H3,(H,9,10) | |
Source | PubChem | |
URL | https://pubchem.ncbi.nlm.nih.gov | |
Description | Data deposited in or computed by PubChem | |
InChI Key |
PCOCFIOYWNCGBM-UHFFFAOYSA-N | |
Source | PubChem | |
URL | https://pubchem.ncbi.nlm.nih.gov | |
Description | Data deposited in or computed by PubChem | |
Canonical SMILES |
CC(C)(C)OC(=O)CCC(=O)O | |
Source | PubChem | |
URL | https://pubchem.ncbi.nlm.nih.gov | |
Description | Data deposited in or computed by PubChem | |
Molecular Formula |
C8H14O4 | |
Source | PubChem | |
URL | https://pubchem.ncbi.nlm.nih.gov | |
Description | Data deposited in or computed by PubChem | |
DSSTOX Substance ID |
DTXSID90399798 | |
Record name | mono-tert-Butyl succinate | |
Source | EPA DSSTox | |
URL | https://comptox.epa.gov/dashboard/DTXSID90399798 | |
Description | DSSTox provides a high quality public chemistry resource for supporting improved predictive toxicology. | |
Molecular Weight |
174.19 g/mol | |
Source | PubChem | |
URL | https://pubchem.ncbi.nlm.nih.gov | |
Description | Data deposited in or computed by PubChem | |
CAS No. |
15026-17-2 | |
Record name | mono-tert-Butyl succinate | |
Source | EPA DSSTox | |
URL | https://comptox.epa.gov/dashboard/DTXSID90399798 | |
Description | DSSTox provides a high quality public chemistry resource for supporting improved predictive toxicology. | |
Record name | 4-(tert-butoxy)-4-oxobutanoic acid | |
Source | European Chemicals Agency (ECHA) | |
URL | https://echa.europa.eu/information-on-chemicals | |
Description | The European Chemicals Agency (ECHA) is an agency of the European Union which is the driving force among regulatory authorities in implementing the EU's groundbreaking chemicals legislation for the benefit of human health and the environment as well as for innovation and competitiveness. | |
Explanation | Use of the information, documents and data from the ECHA website is subject to the terms and conditions of this Legal Notice, and subject to other binding limitations provided for under applicable law, the information, documents and data made available on the ECHA website may be reproduced, distributed and/or used, totally or in part, for non-commercial purposes provided that ECHA is acknowledged as the source: "Source: European Chemicals Agency, http://echa.europa.eu/". Such acknowledgement must be included in each copy of the material. ECHA permits and encourages organisations and individuals to create links to the ECHA website under the following cumulative conditions: Links can only be made to webpages that provide a link to the Legal Notice page. | |
Synthesis routes and methods
Procedure details
Retrosynthesis Analysis
AI-Powered Synthesis Planning: Our tool employs the Template_relevance Pistachio, Template_relevance Bkms_metabolic, Template_relevance Pistachio_ringbreaker, Template_relevance Reaxys, Template_relevance Reaxys_biocatalysis model, leveraging a vast database of chemical reactions to predict feasible synthetic routes.
One-Step Synthesis Focus: Specifically designed for one-step synthesis, it provides concise and direct routes for your target compounds, streamlining the synthesis process.
Accurate Predictions: Utilizing the extensive PISTACHIO, BKMS_METABOLIC, PISTACHIO_RINGBREAKER, REAXYS, REAXYS_BIOCATALYSIS database, our tool offers high-accuracy predictions, reflecting the latest in chemical research and data.
Strategy Settings
Precursor scoring | Relevance Heuristic |
---|---|
Min. plausibility | 0.01 |
Model | Template_relevance |
Template Set | Pistachio/Bkms_metabolic/Pistachio_ringbreaker/Reaxys/Reaxys_biocatalysis |
Top-N result to add to graph | 6 |
Feasible Synthetic Routes
Disclaimer and Information on In-Vitro Research Products
Please be aware that all articles and product information presented on BenchChem are intended solely for informational purposes. The products available for purchase on BenchChem are specifically designed for in-vitro studies, which are conducted outside of living organisms. In-vitro studies, derived from the Latin term "in glass," involve experiments performed in controlled laboratory settings using cells or tissues. It is important to note that these products are not categorized as medicines or drugs, and they have not received approval from the FDA for the prevention, treatment, or cure of any medical condition, ailment, or disease. We must emphasize that any form of bodily introduction of these products into humans or animals is strictly prohibited by law. It is essential to adhere to these guidelines to ensure compliance with legal and ethical standards in research and experimentation.