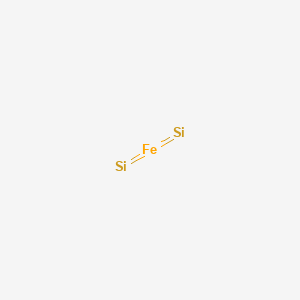
Iron silicide (FeSi2)
Overview
Description
Iron silicide (FeSi2) is an intermetallic compound composed of iron and silicon. It naturally occurs as the rare mineral linzhiite. At room temperature, it forms orthorhombic crystals (β phase), which convert into a tetragonal α phase upon heating to 970°C . Iron silicide is known for its unique electronic and magnetic properties, making it a material of interest in various scientific and industrial applications.
Mechanism of Action
Target of Action
Iron disilicide primarily targets the semiconductor industry and thermoelectric applications . It is used in the fabrication of nanocrystalline thin films and optoelectronic devices . The compound’s primary role is to enhance the performance of these devices due to its unique properties such as high heat and oxidation resistance, and relatively high thermoelectromotive force .
Mode of Action
Iron disilicide interacts with its targets through its semiconducting properties . It forms orthorhombic crystals at room temperature, which convert into a tetragonal α phase upon heating to 970 °C . This transformation is crucial for its application in semiconductor devices .
Biochemical Pathways
physical and engineering processes of semiconductor fabrication. Its interaction with silicon in the formation of nanocrystalline thin films is a key process in the manufacturing of optoelectronic devices .
Pharmacokinetics
The term pharmacokinetics typically applies to drugs and their absorption, distribution, metabolism, and excretion (ADME) in the bodyformation, application, transformation, and disposal . Iron disilicide is formed through processes like ion-beam synthesis and solid-state reaction . Its transformation under different temperatures and its interaction with silicon are crucial for its application .
Result of Action
The result of iron disilicide’s action is the creation of high-performance semiconductor devices . Its semiconducting properties, combined with its stability and resistance to heat and oxidation, make it an excellent material for enhancing the performance of these devices . In addition, it has been shown to produce hydrogen by the action of sodium hydroxide solution on silicon, with iron disilicide acting as a spectator/protector species for the silicon .
Action Environment
The action of iron disilicide is influenced by environmental factors such as temperature and chemical environment . Its transformation from orthorhombic to tetragonal phase occurs at a specific temperature , and its interaction with other elements, such as silicon, is crucial for its application in semiconductor devices .
Preparation Methods
Synthetic Routes and Reaction Conditions: Iron silicide can be synthesized through several methods, including solid-state reactions, sputtering, and chemical vapor deposition. One common method involves the reaction of iron sesquioxide (Fe2O3) with elemental silicon and active metals such as magnesium or lithium at 650°C in a stainless steel autoclave . Another method involves the deposition of iron and silicon multilayers on a silicon substrate, followed by thermal annealing at temperatures ranging from 600°C to 1000°C .
Industrial Production Methods: In industrial settings, iron silicide is often produced using high-temperature reduction processes. For example, the reduction of iron oxide with silicon in an electric arc furnace can yield iron silicide. Additionally, the use of magnetron sputtering deposition under a vacuum environment allows for the production of thin films of iron silicide, which are used in various electronic applications .
Chemical Reactions Analysis
Types of Reactions: Iron silicide undergoes several types of chemical reactions, including oxidation, reduction, and substitution.
Common Reagents and Conditions:
Oxidation: Iron silicide can be oxidized in the presence of oxygen at elevated temperatures, forming iron oxide and silicon dioxide.
Reduction: The reduction of iron silicide can be achieved using reducing agents such as hydrogen or carbon monoxide.
Substitution: Iron silicide can undergo substitution reactions with other metals, leading to the formation of various metal silicides.
Major Products Formed:
Oxidation: Iron oxide (Fe2O3) and silicon dioxide (SiO2).
Reduction: Elemental iron (Fe) and silicon (Si).
Substitution: Formation of other metal silicides, such as cobalt silicide (CoSi2) and manganese silicide (MnSi2).
Scientific Research Applications
Iron silicide has a wide range of scientific research applications due to its unique properties:
Chemistry: Iron silicide is used as a catalyst in various chemical reactions, including hydrogenation and dehydrogenation processes.
Biology: Research is ongoing to explore the potential use of iron silicide nanoparticles in biomedical applications, such as drug delivery and imaging.
Medicine: Iron silicide’s magnetic properties make it a candidate for use in magnetic resonance imaging (MRI) contrast agents.
Industry: Iron silicide is used in the production of high-performance solar cells, where it serves as an absorber layer to enhance photovoltaic efficiency
Comparison with Similar Compounds
- Cobalt silicide (CoSi2)
- Manganese silicide (MnSi2)
- Titanium silicide (TiSi2)
- Magnesium silicide (Mg2Si)
Iron silicide’s combination of electronic and magnetic properties, along with its versatility in various applications, makes it a compound of significant interest in both scientific research and industrial applications.
Properties
InChI |
InChI=1S/Fe.2Si | |
---|---|---|
Source | PubChem | |
URL | https://pubchem.ncbi.nlm.nih.gov | |
Description | Data deposited in or computed by PubChem | |
InChI Key |
JRACIMOSEUMYIP-UHFFFAOYSA-N | |
Source | PubChem | |
URL | https://pubchem.ncbi.nlm.nih.gov | |
Description | Data deposited in or computed by PubChem | |
Canonical SMILES |
[Si]=[Fe]=[Si] | |
Source | PubChem | |
URL | https://pubchem.ncbi.nlm.nih.gov | |
Description | Data deposited in or computed by PubChem | |
Molecular Formula |
FeSi2 | |
Source | PubChem | |
URL | https://pubchem.ncbi.nlm.nih.gov | |
Description | Data deposited in or computed by PubChem | |
DSSTOX Substance ID |
DTXSID00892427 | |
Record name | Iron bisilicide | |
Source | EPA DSSTox | |
URL | https://comptox.epa.gov/dashboard/DTXSID00892427 | |
Description | DSSTox provides a high quality public chemistry resource for supporting improved predictive toxicology. | |
Molecular Weight |
112.02 g/mol | |
Source | PubChem | |
URL | https://pubchem.ncbi.nlm.nih.gov | |
Description | Data deposited in or computed by PubChem | |
CAS No. |
12022-99-0 | |
Record name | Iron silicide (FeSi2) | |
Source | CAS Common Chemistry | |
URL | https://commonchemistry.cas.org/detail?cas_rn=12022-99-0 | |
Description | CAS Common Chemistry is an open community resource for accessing chemical information. Nearly 500,000 chemical substances from CAS REGISTRY cover areas of community interest, including common and frequently regulated chemicals, and those relevant to high school and undergraduate chemistry classes. This chemical information, curated by our expert scientists, is provided in alignment with our mission as a division of the American Chemical Society. | |
Explanation | The data from CAS Common Chemistry is provided under a CC-BY-NC 4.0 license, unless otherwise stated. | |
Record name | Iron silicide (FeSi2) | |
Source | ChemIDplus | |
URL | https://pubchem.ncbi.nlm.nih.gov/substance/?source=chemidplus&sourceid=0012022990 | |
Description | ChemIDplus is a free, web search system that provides access to the structure and nomenclature authority files used for the identification of chemical substances cited in National Library of Medicine (NLM) databases, including the TOXNET system. | |
Record name | Iron silicide (FeSi2) | |
Source | EPA Chemicals under the TSCA | |
URL | https://www.epa.gov/chemicals-under-tsca | |
Description | EPA Chemicals under the Toxic Substances Control Act (TSCA) collection contains information on chemicals and their regulations under TSCA, including non-confidential content from the TSCA Chemical Substance Inventory and Chemical Data Reporting. | |
Record name | Iron bisilicide | |
Source | EPA DSSTox | |
URL | https://comptox.epa.gov/dashboard/DTXSID00892427 | |
Description | DSSTox provides a high quality public chemistry resource for supporting improved predictive toxicology. | |
Record name | Iron disilicide | |
Source | European Chemicals Agency (ECHA) | |
URL | https://echa.europa.eu/substance-information/-/substanceinfo/100.031.507 | |
Description | The European Chemicals Agency (ECHA) is an agency of the European Union which is the driving force among regulatory authorities in implementing the EU's groundbreaking chemicals legislation for the benefit of human health and the environment as well as for innovation and competitiveness. | |
Explanation | Use of the information, documents and data from the ECHA website is subject to the terms and conditions of this Legal Notice, and subject to other binding limitations provided for under applicable law, the information, documents and data made available on the ECHA website may be reproduced, distributed and/or used, totally or in part, for non-commercial purposes provided that ECHA is acknowledged as the source: "Source: European Chemicals Agency, http://echa.europa.eu/". Such acknowledgement must be included in each copy of the material. ECHA permits and encourages organisations and individuals to create links to the ECHA website under the following cumulative conditions: Links can only be made to webpages that provide a link to the Legal Notice page. | |
Q1: What is the molecular formula and weight of iron disilicide?
A1: The molecular formula for iron disilicide is FeSi2. Its molecular weight is 119.88 g/mol.
Q2: What are the different phases of iron disilicide and what makes the beta phase particularly interesting for thermoelectric applications?
A2: Iron disilicide exists in several phases, including α-FeSi2, β-FeSi2, and others. The β-FeSi2 phase is a semiconductor with a direct band gap of ~0.85-0.89 eV [, ], making it suitable for potential applications in thermoelectric devices, optical communication, and detectors.
Q3: What are some techniques used to characterize the structure and properties of iron disilicide?
A3: Researchers employ various techniques to characterize iron disilicide, including:
- X-ray diffraction (XRD): Identifies different phases and determines crystal structure [, , , , , , , , , , , ].
- Scanning Electron Microscopy (SEM): Provides information about the morphology and microstructure [, , , , ].
- Transmission Electron Microscopy (TEM): Allows for high-resolution imaging of the nanostructure and defects [, ].
- Electron Paramagnetic Resonance (EPR): Investigates point defects within the material [, ].
- Optical spectroscopy (UV-Vis-NIR): Determines optical properties like band gap and absorption coefficient [, , , , ].
- Electrical measurements (resistivity, Hall effect): Characterize the electrical properties and determine the type of conductivity (n-type or p-type) [, , , , , , , , , ].
- Thermoelectric measurements (Seebeck coefficient, thermal conductivity): Evaluate the material's efficiency for thermoelectric applications [, , , , , , , , , ].
Q4: What are some common methods for synthesizing iron disilicide?
A4: Several methods exist for synthesizing FeSi2, each with advantages and drawbacks:
- Mechanical alloying: Involves high-energy ball milling of elemental powders, resulting in nanocomposite powders with reduced particle size, enhancing thermoelectric properties [, , ].
- Sintering: Consolidates powders into dense ceramics using heat and pressure. This can be combined with doping strategies to tune electrical properties [, , , ].
- Rapid solidification: Creates fine-grained materials by rapidly cooling molten alloys, often followed by hot pressing for densification [, , ].
- Chemical Vapor Deposition (CVD): Deposits thin films of FeSi2 onto substrates. This method allows for precise control over film thickness and composition [, , , , ].
- Pulsed Laser Deposition (PLD): Uses a high-power laser to ablate a target material, depositing thin films onto a substrate. This is particularly useful for producing high-quality, controlled stoichiometry thin films [, , ].
- Ion Beam Synthesis (IBS): Employs ion implantation to introduce iron into a silicon substrate, followed by annealing to form iron disilicide layers [, ].
Q5: What are the challenges in synthesizing pure β-FeSi2, and how do researchers overcome them?
A5: Obtaining phase-pure β-FeSi2 can be challenging due to the formation of other phases like α-FeSi2 and FeSi. Researchers use various strategies to promote β-FeSi2 formation:
- Precise control of stoichiometry: Maintaining the correct Fe:Si ratio is crucial during synthesis [, , , , ].
- Post-synthesis annealing: Annealing at specific temperatures and durations can convert other phases to β-FeSi2 and improve crystallinity [, , , , , , ].
- Doping with additional elements: Introducing dopants like Al, Mn, Co, or Cu can stabilize the β-FeSi2 phase and tailor its thermoelectric properties [, , , , , , ].
Q6: How does doping affect the properties of iron disilicide?
A6: Doping is a common strategy to optimize FeSi2's thermoelectric properties:
- Al doping: Typically results in p-type conductivity and can enhance the Seebeck coefficient while reducing thermal conductivity [, , , , , ].
- Mn doping: Can lead to p-type conductivity and increase the figure of merit by influencing carrier concentration and mobility [, , , , ].
- Co doping: Generally produces n-type conductivity and has been shown to enhance the figure of merit by modifying the local composition and reducing thermal conductivity [, ].
- Other dopants: Elements like Cr, Nd, Ru, Rh, and Pd have also been investigated for their influence on the electrical and thermoelectric properties of FeSi2 [, ].
Q7: What are the potential applications of iron disilicide?
A7: FeSi2 holds promise for various applications due to its unique properties:
- Thermoelectric generators: β-FeSi2 is being explored as a material for converting waste heat into electricity due to its semiconducting nature and non-toxicity [, , , , , ].
- Solar cells: The direct bandgap of β-FeSi2 makes it suitable for thin-film solar cell applications, particularly in combination with silicon [, , , , , , ].
- Optoelectronics: FeSi2's optical properties make it a potential candidate for developing light-emitting diodes (LEDs) and photodetectors [, , , , ].
Q8: What are the current limitations and future research directions for iron disilicide?
A8: Despite its potential, several challenges remain for FeSi2:
- Improving thermoelectric efficiency: While promising, the figure of merit of FeSi2 needs further improvement for practical thermoelectric applications [, ].
- Controlling defect formation: Defects can significantly impact the electrical and thermoelectric properties of FeSi2. Further research is needed to understand and control their formation and influence [, ].
- Scalable and cost-effective production: Developing scalable and cost-effective fabrication methods for high-quality FeSi2 is crucial for commercialization [].
Disclaimer and Information on In-Vitro Research Products
Please be aware that all articles and product information presented on BenchChem are intended solely for informational purposes. The products available for purchase on BenchChem are specifically designed for in-vitro studies, which are conducted outside of living organisms. In-vitro studies, derived from the Latin term "in glass," involve experiments performed in controlled laboratory settings using cells or tissues. It is important to note that these products are not categorized as medicines or drugs, and they have not received approval from the FDA for the prevention, treatment, or cure of any medical condition, ailment, or disease. We must emphasize that any form of bodily introduction of these products into humans or animals is strictly prohibited by law. It is essential to adhere to these guidelines to ensure compliance with legal and ethical standards in research and experimentation.