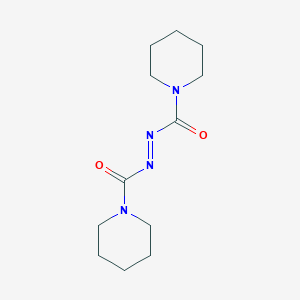
1,1'-(Azodicarbonyl)dipiperidine
- Click on QUICK INQUIRY to receive a quote from our team of experts.
- With the quality product at a COMPETITIVE price, you can focus more on your research.
Overview
Description
1,1’-(Azodicarbonyl)dipiperidine, also known as azodicarboxylic acid dipiperidide, is a chemical compound with the molecular formula C12H20N4O2 and a molecular weight of 252.31 g/mol . This compound is characterized by its azo and carbonyl functional groups and is widely used as a reagent in organic synthesis, particularly in the Mitsunobu reaction .
Scientific Research Applications
1,1’-(Azodicarbonyl)dipiperidine has a wide range of applications in scientific research:
Mechanism of Action
Target of Action
It has been used as a reagent in the synthesis of g protein-coupled receptor 120 (gpr120) agonists and peroxisome proliferator-activated receptor α (PPARα), PPARγ, and PPARδ triple agonists . These receptors play crucial roles in metabolic regulation.
Mode of Action
AdDP is a widely employed reagent in the Mitsunobu reaction , which is a chemical reaction that converts an alcohol into a variety of functional groups, such as esters, amides, and ethers . It facilitates the condensation between an alcohol and an acidic compound .
Biochemical Pathways
AdDP is involved in the synthesis of various compounds through the Mitsunobu reaction . It has been used in the preparation of polyfluoroalkylated tripyrazolylmethane ligands, optically active α,α-disubstituted amino acids, aza-β-lactams through [2+2] cycloaddition reactions, glycosyl disulfides, pyridine ether PPAR agonists, and S-glycosyl amino acid building blocks for combinatorial neoglycopeptide synthesis .
Pharmacokinetics
It is soluble in organic solvents such as ethanol and dimethylformamide (dmf), with solubilities of approximately 1 mg/ml and 10 mg/ml, respectively . This solubility profile may influence its bioavailability.
Result of Action
The result of AdDP’s action largely depends on its use in the synthesis of other compounds. For instance, when used in the synthesis of GPR120 agonists, it can contribute to antidiabetic activity . Similarly, in the synthesis of PPARα, PPARγ, and PPARδ triple agonists, it can contribute to the regulation of lipid and glucose metabolism .
Action Environment
Environmental factors such as temperature, pH, and the presence of other chemicals can influence the efficacy and stability of AdDP. For instance, the Mitsunobu reaction, in which AdDP is commonly used, requires anhydrous conditions and is typically performed at room temperature . Additionally, the solubility of AdDP in different solvents can affect its reactivity .
Safety and Hazards
Biochemical Analysis
Biochemical Properties
1,1’-(Azodicarbonyl)dipiperidine is involved in various biochemical reactions. It has been used as a reagent in the synthesis of G protein-coupled receptor 120 (GPR120) agonists with antidiabetic activity and peroxisome proliferator-activated receptor α (PPARα), PPARγ, and PPARδ triple agonists
Cellular Effects
Given its role in the synthesis of GPR120 agonists and PPAR activators , it may indirectly influence cell function by modulating these signaling pathways. GPR120 and PPARs are involved in various cellular processes, including inflammation, lipid metabolism, and glucose homeostasis .
Molecular Mechanism
The molecular mechanism of 1,1’-(Azodicarbonyl)dipiperidine primarily involves its role as a reagent in the Mitsunobu reaction . In this reaction, it facilitates the coupling of two different functional groups or the inversion of stereochemistry of alcohols . The exact mechanism at the molecular level depends on the specific reaction conditions and the other reactants involved.
Temporal Effects in Laboratory Settings
It is known to be a solid at room temperature and has a melting point of 134-136 °C .
Preparation Methods
Synthetic Routes and Reaction Conditions: 1,1’-(Azodicarbonyl)dipiperidine can be synthesized through the reaction of azodicarboxylic acid with piperidine under controlled conditions . The reaction typically involves the use of solvents such as ethanol or dimethylformamide (DMF) and requires purging with an inert gas to prevent oxidation . The compound is then purified through recrystallization to obtain a high-purity product.
Industrial Production Methods: In industrial settings, the production of 1,1’-(Azodicarbonyl)dipiperidine follows similar synthetic routes but on a larger scale. The process involves the use of automated reactors and continuous flow systems to ensure consistent quality and yield . The final product is subjected to rigorous quality control measures to meet industry standards.
Chemical Reactions Analysis
Types of Reactions: 1,1’-(Azodicarbonyl)dipiperidine undergoes various chemical reactions, including:
Oxidation: The compound can be oxidized to form corresponding oxides.
Reduction: Reduction reactions can convert the azo group to hydrazine derivatives.
Common Reagents and Conditions:
Oxidation: Common oxidizing agents include hydrogen peroxide and potassium permanganate.
Reduction: Reducing agents such as sodium borohydride or lithium aluminum hydride are used.
Substitution: The Mitsunobu reaction typically involves the use of triphenylphosphine and diethyl azodicarboxylate under anhydrous conditions.
Major Products:
Oxidation: Formation of oxides and related compounds.
Reduction: Formation of hydrazine derivatives.
Substitution: Formation of esters, ethers, and other derivatives through the Mitsunobu reaction.
Comparison with Similar Compounds
Diethyl azodicarboxylate: Another commonly used reagent in the Mitsunobu reaction.
Azodicarboxylic acid diethyl ester: Similar in structure and reactivity to 1,1’-(Azodicarbonyl)dipiperidine.
Uniqueness: 1,1’-(Azodicarbonyl)dipiperidine is unique due to its specific structural features, which provide distinct reactivity and selectivity in organic synthesis . Its ability to facilitate the Mitsunobu reaction with high efficiency makes it a valuable reagent in both academic and industrial research .
Properties
CAS No. |
10465-81-3 |
---|---|
Molecular Formula |
C12H20N4O2 |
Molecular Weight |
252.31 g/mol |
IUPAC Name |
N-(piperidine-1-carbonylimino)piperidine-1-carboxamide |
InChI |
InChI=1S/C12H20N4O2/c17-11(15-7-3-1-4-8-15)13-14-12(18)16-9-5-2-6-10-16/h1-10H2 |
InChI Key |
OQJBFFCUFALWQL-UHFFFAOYSA-N |
Isomeric SMILES |
C1CCN(CC1)C(=O)/N=N/C(=O)N2CCCCC2 |
SMILES |
C1CCN(CC1)C(=O)N=NC(=O)N2CCCCC2 |
Canonical SMILES |
C1CCN(CC1)C(=O)N=NC(=O)N2CCCCC2 |
10465-81-3 | |
Pictograms |
Irritant |
Synonyms |
diazenedicarboxylic acid bis(N,N-piperidide) SR 4077 SR-4077 |
Origin of Product |
United States |
Retrosynthesis Analysis
AI-Powered Synthesis Planning: Our tool employs the Template_relevance Pistachio, Template_relevance Bkms_metabolic, Template_relevance Pistachio_ringbreaker, Template_relevance Reaxys, Template_relevance Reaxys_biocatalysis model, leveraging a vast database of chemical reactions to predict feasible synthetic routes.
One-Step Synthesis Focus: Specifically designed for one-step synthesis, it provides concise and direct routes for your target compounds, streamlining the synthesis process.
Accurate Predictions: Utilizing the extensive PISTACHIO, BKMS_METABOLIC, PISTACHIO_RINGBREAKER, REAXYS, REAXYS_BIOCATALYSIS database, our tool offers high-accuracy predictions, reflecting the latest in chemical research and data.
Strategy Settings
Precursor scoring | Relevance Heuristic |
---|---|
Min. plausibility | 0.01 |
Model | Template_relevance |
Template Set | Pistachio/Bkms_metabolic/Pistachio_ringbreaker/Reaxys/Reaxys_biocatalysis |
Top-N result to add to graph | 6 |
Feasible Synthetic Routes
Disclaimer and Information on In-Vitro Research Products
Please be aware that all articles and product information presented on BenchChem are intended solely for informational purposes. The products available for purchase on BenchChem are specifically designed for in-vitro studies, which are conducted outside of living organisms. In-vitro studies, derived from the Latin term "in glass," involve experiments performed in controlled laboratory settings using cells or tissues. It is important to note that these products are not categorized as medicines or drugs, and they have not received approval from the FDA for the prevention, treatment, or cure of any medical condition, ailment, or disease. We must emphasize that any form of bodily introduction of these products into humans or animals is strictly prohibited by law. It is essential to adhere to these guidelines to ensure compliance with legal and ethical standards in research and experimentation.