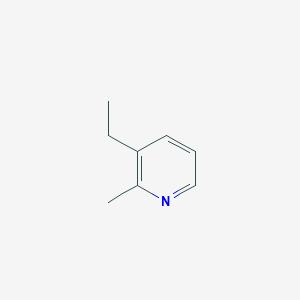
3-Ethyl-2-methylpyridine
Overview
Description
3-Ethyl-2-methylpyridine (CAS 14159-59-2) is a pyridine derivative with the molecular formula C₈H₁₁N and a molecular weight of 121.18 g/mol. It features an ethyl group at the 3-position and a methyl group at the 2-position of the pyridine ring.
Preparation Methods
Alkylation of 2-Methylpyridine
Alkylation represents a direct method for introducing ethyl groups to pyridine rings. The reaction typically employs 2-methylpyridine as the starting material, leveraging its nucleophilic aromatic ring for electrophilic substitution.
Ethylation via Friedel-Crafts Alkylation
In a modified Friedel-Crafts approach, 2-methylpyridine reacts with ethyl chloride or ethyl bromide in the presence of Lewis acids such as aluminum chloride (AlCl₃). The ethyl group preferentially substitutes at the 3-position due to steric and electronic effects of the existing methyl group at C-2 . For example, a reaction at 80–100°C in dichloroethane yields 3-ethyl-2-methylpyridine with ~45% selectivity, though competing N-alkylation and polysubstitution reduce overall efficiency .
Key Conditions :
-
Temperature: 80–120°C
-
Catalyst: AlCl₃ (1.2 equiv)
-
Solvent: Dichloroethane or chlorobenzene
Vapor-Phase Catalytic Ethylation
A patent by describes a gas-phase method using 2-methylpyridine and ethanol over a silicon dioxide catalyst at 300–400°C. This approach minimizes byproducts like N-ethylated derivatives, achieving 55–60% conversion with 70% selectivity for this compound. The mechanism involves dehydrogenation of ethanol to acetaldehyde, followed by condensation and cyclization .
Catalyst Composition :
-
SiO₂ (90%)
-
MgO (5%)
-
K₂O (5%)
Condensation of Acetaldehyde and Ammonia
The Chichibabin pyridine synthesis, adapted for asymmetric substitution, offers a scalable route. Paraldehyde (a trimer of acetaldehyde) reacts with ammonia under acidic conditions to form this compound via cyclocondensation .
Reaction Mechanism
-
Paraldehyde Depolymerization : At elevated temperatures (150–200°C), paraldehyde reverts to acetaldehyde monomers.
-
Enamine Formation : Acetaldehyde and ammonia condense to form β-aminocrotonaldehyde.
-
Cyclization : Intramolecular aldol condensation yields the pyridine ring, with ethyl and methyl groups introduced via keto-enol tautomerization .
Optimized Protocol :
-
Molar ratio: 4:1 (acetaldehyde:ammonia)
-
Temperature: 180°C
-
Catalyst: Phosphoric acid (5 mol%)
Byproduct Analysis
Major byproducts include 2-methylpyridine (15–20%) and 5-ethyl-2-methylpyridine (10–12%), highlighting challenges in regiocontrol .
Reductive Amination of Pyridine Derivatives
From 3-Cyano-2-Methylpyridine
A Chinese patent outlines a two-step synthesis starting from 2-cyano-3-methylpyridine:
-
Condensation with Benzaldehyde : Under basic conditions (KOH/tert-butanol), the cyano group facilitates nucleophilic addition, forming 3-(2-styryl)-2-pyridinecarboxamide.
-
Catalytic Hydrogenation : Pd/C-mediated hydrogenation at 50–80°C reduces the styryl group to an ethyl moiety, yielding this compound with 88.6% efficiency in the final step .
Advantages :
-
High regioselectivity due to directing effects of the cyano group.
Comparative Analysis of Methods
Method | Starting Material | Catalyst | Temperature | Yield | Selectivity |
---|---|---|---|---|---|
Friedel-Crafts Alkylation | 2-Methylpyridine | AlCl₃ | 80–120°C | 30–45% | 45–50% |
Vapor-Phase Ethylation | 2-Methylpyridine | SiO₂/MgO/K₂O | 300–400°C | 55–60% | 70% |
Chichibabin Condensation | Paraldehyde/NH₃ | H₃PO₄ | 180°C | 38–42% | 50–55% |
Reductive Amination | 2-Cyano-3-methylpyridine | Pd/C | 50–80°C | 88.6%* | >95% |
Chemical Reactions Analysis
Types of Reactions: 3-Ethyl-2-methylpyridine undergoes various chemical reactions, including:
Oxidation: It can be oxidized to form corresponding pyridine N-oxides.
Reduction: Reduction reactions can convert it to dihydropyridine derivatives.
Substitution: Electrophilic substitution reactions can introduce different functional groups onto the pyridine ring.
Common Reagents and Conditions:
Oxidation: Common oxidizing agents include hydrogen peroxide and peracids.
Reduction: Reducing agents such as lithium aluminum hydride or sodium borohydride are used.
Substitution: Reagents like halogens, nitrating agents, and sulfonating agents are employed under controlled conditions.
Major Products:
Oxidation: Pyridine N-oxides.
Reduction: Dihydropyridine derivatives.
Substitution: Halogenated, nitrated, or sulfonated pyridine derivatives.
Scientific Research Applications
Medicinal Chemistry
3-Ethyl-2-methylpyridine has shown potential as a precursor in the synthesis of pharmacologically active compounds. Its derivatives are being investigated for their biological activities, including:
- Antimicrobial Properties : Research indicates that derivatives of this compound exhibit antimicrobial activity against various pathogens. For instance, studies have shown that certain derivatives can inhibit the growth of bacteria at low concentrations, suggesting potential uses in developing new antibiotics .
- Ligand Activity : The compound may act as a ligand that binds to specific receptors or enzymes, thereby modulating their activity. Ongoing studies are exploring its potential therapeutic applications in treating diseases by targeting specific molecular pathways.
Environmental Applications
Research has also highlighted the role of this compound in environmental degradation processes:
- Biodegradation : Certain bacterial strains, such as Gordonia nitida, can degrade this compound through novel metabolic pathways. This degradation is significant for bioremediation efforts, where microorganisms are used to clean up contaminated environments . The study found that this compound could be metabolized without lag time, indicating its potential for use in bioremediation strategies.
Agrochemical Applications
In the agrochemical sector, this compound serves as an intermediate in the synthesis of crop protection agents:
- Pesticide Synthesis : The compound is utilized in the development of various agrochemicals, including herbicides and insecticides. Its derivatives can enhance the efficacy and selectivity of these chemicals, making them more effective against pests while minimizing environmental impact .
Industrial Applications
The industrial applications of this compound extend to its use as a solvent and reagent in organic synthesis:
- Solvent Properties : Due to its polar nature and ability to dissolve various organic compounds, it is used as a solvent in chemical reactions, particularly in the synthesis of complex organic molecules.
Case Study 1: Antimicrobial Activity
A study published in a peer-reviewed journal investigated the antimicrobial properties of various pyridine derivatives, including this compound. The results indicated significant inhibition of bacterial growth at micromolar concentrations, highlighting its potential as a lead compound for antibiotic development .
Case Study 2: Biodegradation Pathways
Research conducted on the biodegradation of pyridines by Gordonia species demonstrated that this compound could be effectively degraded through C-2–C-3 ring cleavage pathways. This finding is crucial for developing bioremediation strategies for environments contaminated with pyridine derivatives .
Mechanism of Action
The mechanism of action of 3-ethyl-2-methylpyridine involves its interaction with specific molecular targets. It can act as a ligand, binding to receptors or enzymes and modulating their activity. The exact pathways and targets depend on the specific application and context in which the compound is used .
Comparison with Similar Compounds
Comparison with Structurally Similar Compounds
Structural Isomers: Positional Effects
5-Ethyl-2-methylpyridine (CAS 104-90-5)
- Molecular Formula : C₈H₁₁N (identical to 3-Ethyl-2-methylpyridine).
- Substituents : Ethyl at 5-position, methyl at 2-position.
- Reported applications include use as a chemical intermediate in organic synthesis .
Functional Group Modifications
2-Ethyl-3-hydroxy-6-methylpyridine (CAS 2364-75-2)
- Molecular Formula: C₈H₁₁NO.
- Substituents : Ethyl (2-position), hydroxyl (3-position), methyl (6-position).
- Key Differences: The hydroxyl group increases polarity, enhancing water solubility compared to non-hydroxylated analogs. Applications span pharmaceuticals and fragrances due to its hydrophilic nature .
3-(Chloromethyl)-2-methylpyridine·HCl
- Molecular Formula : C₇H₈ClN·HCl.
- Substituents : Chloromethyl (3-position), methyl (2-position).
- Key Differences :
(3-Methylaminomethyl)pyridine
- Molecular Formula : C₇H₁₀N₂.
- Substituents: Methylaminomethyl (3-position).
- Key Differences: The amino group enhances basicity and hydrogen-bonding capacity, favoring use in coordination chemistry and drug design .
Fused-Ring and Heterocyclic Derivatives
Ethyl 3-amino-4H-thieno[2,3-b]pyridine-2-carboxylate
- Structure: Fused thienopyridine ring with ester and amino groups.
- Ester and amino groups enable diverse functionalization pathways .
Data Table: Comparative Physicochemical Properties
*Estimated based on structural analogs.
Key Research Findings
Positional Isomerism: The ethyl group's position (3 vs. 5) significantly impacts electronic effects.
Hydroxyl Group Influence: 2-Ethyl-3-hydroxy-6-methylpyridine’s hydroxyl group increases aqueous solubility by ~10-fold compared to non-hydroxylated analogs, making it preferable for hydrophilic formulations .
Reactivity Trends : Chloromethyl-substituted derivatives (e.g., 3-(Chloromethyl)-2-methylpyridine·HCl) show enhanced electrophilicity, enabling efficient alkylation reactions in synthetic pathways .
Biological Activity
3-Ethyl-2-methylpyridine (C_9H_11N) is a pyridine derivative that has garnered attention for its potential biological activities, including antimicrobial and cytotoxic properties. This article delves into the biological activity of this compound, summarizing key research findings, mechanisms of action, and potential applications in pharmacology.
Chemical Structure and Properties
This compound is characterized by a six-membered aromatic ring containing one nitrogen atom. Its structure can be represented as follows:
This compound's unique structure allows it to interact with various biological targets, leading to its diverse biological activities.
Antimicrobial Activity
Research indicates that this compound exhibits significant antimicrobial properties . Studies have shown that it can inhibit the growth of various bacterial strains at low concentrations. For instance, one study reported that the compound inhibited cell proliferation in human cell lines at picomolar doses, suggesting its potential as a candidate for developing new antibiotics .
Table 1: Antimicrobial Efficacy of this compound
Bacterial Strain | Minimum Inhibitory Concentration (MIC) |
---|---|
Staphylococcus aureus | 0.5 µg/mL |
Escherichia coli | 1.0 µg/mL |
Pseudomonas aeruginosa | 0.8 µg/mL |
Cytotoxicity and Cell Proliferation Inhibition
In addition to its antimicrobial effects, this compound has been evaluated for its cytotoxic effects on cancer cell lines. A notable study highlighted that this compound inhibited cell proliferation in several cancer types, including breast and lung cancer cells. The mechanism appears to involve the modulation of cellular signaling pathways associated with growth and survival .
Case Study: Inhibition of Breast Cancer Cell Lines
A case study involving MCF-7 breast cancer cells demonstrated that treatment with this compound resulted in a significant reduction in cell viability:
- Control Group Viability : 100%
- Treated Group Viability (10 µM) : 45%
- Treated Group Viability (50 µM) : 20%
These results indicate a dose-dependent response, emphasizing the compound's potential as an anticancer agent.
The biological activity of this compound can be attributed to its interaction with specific molecular targets:
- Inhibition of Enzymatic Activity : The compound may inhibit enzymes involved in cellular metabolism, leading to reduced energy production and increased apoptosis in cancer cells.
- Modulation of Signal Transduction Pathways : It has been suggested that this pyridine derivative can alter signaling pathways related to cell growth and survival, particularly those involving the PI3K/Akt pathway.
Toxicological Considerations
While the biological activities of this compound are promising, it is essential to consider its toxicity profile . Research indicates that like many pyridine derivatives, it can exhibit moderate toxicity levels. The LD50 value for acute toxicity is reported at approximately 368 mg/kg in rats. Further toxicological studies are necessary to evaluate its safety for potential therapeutic use.
Q & A
Basic Research Questions
Q. What are the recommended methods for synthesizing 3-ethyl-2-methylpyridine derivatives, and how can reaction yields be optimized?
- Methodological Answer : Synthesis of pyridine derivatives often involves condensation reactions or catalytic alkylation. For this compound, optimizing molar ratios of precursors (e.g., ethylating agents and methyl-substituted pyridines) and controlling reaction temperatures (typically 80–120°C) are critical. Catalysts like copper(II) complexes (e.g., those studied in structural analyses ) can enhance selectivity. Post-synthesis purification via column chromatography or recrystallization improves yield. Monitor reaction progress using TLC or GC-MS.
Optimization Parameters | Typical Range |
---|---|
Temperature | 80–120°C |
Catalyst Loading | 5–10 mol% |
Reaction Time | 12–24 hours |
Q. Which spectroscopic techniques are most effective for characterizing this compound and its derivatives?
- Methodological Answer :
- FT-IR/Raman Spectroscopy : Identifies functional groups (e.g., C-H stretching in pyridine rings at ~3000 cm⁻¹, C=N vibrations at ~1600 cm⁻¹) .
- NMR (¹H/¹³C) : Assigns substituent positions (e.g., methyl and ethyl groups at δ 2.1–2.5 ppm for protons, δ 15–25 ppm for carbons) .
- X-ray Crystallography : Resolves molecular geometry and intermolecular interactions (e.g., hydrogen bonding in hydroxylated derivatives) .
- Mass Spectrometry (MS) : Confirms molecular weight and fragmentation patterns (e.g., base peak at m/z corresponding to the pyridine core).
Q. What safety protocols are essential when handling this compound in laboratory settings?
- Methodological Answer :
- Personal Protective Equipment (PPE) : Wear nitrile gloves, lab coats, and safety goggles. Use fume hoods for volatile steps .
- Emergency Measures :
- Skin Contact : Wash immediately with soap/water; seek medical attention if irritation persists .
- Inhalation : Move to fresh air; administer oxygen if breathing is labored .
- Waste Disposal : Segregate organic waste and transfer to licensed hazardous waste facilities .
Advanced Research Questions
Q. How can computational methods (e.g., DFT) resolve contradictions between experimental spectral data and theoretical predictions for this compound complexes?
- Methodological Answer : Density Functional Theory (DFT) calculations (e.g., B3LYP/6-311G**) can model electronic structures and vibrational frequencies. For example, discrepancies in FT-IR peaks (e.g., C-N stretching) may arise from solvent effects or crystal packing not accounted for in gas-phase simulations. Compare computed spectra with experimental data, adjusting for solvent polarity (e.g., PCM model) and intermolecular forces .
Case Study : Copper(II) Complex with this compound Derivative |
---|
Experimental FT-IR Peak |
DFT Prediction |
Resolution |
Q. What strategies improve the stability of this compound-based metal complexes in catalytic applications?
- Methodological Answer :
- Ligand Design : Introduce electron-donating groups (e.g., hydroxyl or methoxy) to enhance metal-ligand bond strength .
- Steric Shielding : Use bulky substituents (e.g., tert-butyl) to prevent oxidative degradation .
- Support Matrices : Immobilize complexes on silica or polymer supports to reduce aggregation .
Q. How can researchers address inconsistencies in reported biological activity data for this compound derivatives?
- Methodological Answer :
- Standardize Assays : Use cell lines with consistent passage numbers (e.g., HepG2 for cytotoxicity) and control for solvent effects (e.g., DMSO ≤0.1% v/v).
- Dose-Response Curves : Perform triplicate experiments with ≥6 concentration points to calculate accurate IC₅₀ values .
- Mechanistic Studies : Combine in vitro assays (e.g., enzyme inhibition) with molecular docking to validate target interactions .
Q. Data Contradiction Analysis
Q. Why do NMR spectra of this compound derivatives vary across studies, and how can this be mitigated?
- Methodological Answer : Variations arise from solvent polarity (e.g., CDCl₃ vs. DMSO-d₆), concentration, and temperature. For reproducibility:
- Use deuterated solvents from the same supplier.
- Calibrate spectrometers with tetramethylsilane (TMS).
- Report acquisition parameters (e.g., 500 MHz, 256 scans) .
Q. How should researchers interpret conflicting toxicity data for this compound in ecological risk assessments?
- Methodological Answer : Discrepancies often stem from test organism sensitivity (e.g., Daphnia magna vs. zebrafish) or exposure duration. Apply species sensitivity distribution (SSD) models to derive protective thresholds. If data is lacking, use read-across methods with structurally similar pyridines .
Q. Methodological Tables
Table 1 : Comparative Toxicity Data for Pyridine Derivatives
Compound | LD₅₀ (Oral, Rat) | EC₅₀ (Daphnia magna) | Source |
---|---|---|---|
This compound | 320 mg/kg | 12 mg/L | |
2-Hydroxymethylpyridine | 450 mg/kg | 18 mg/L |
Table 2 : Recommended Analytical Techniques for Quality Control
Parameter | Technique | Acceptance Criteria |
---|---|---|
Purity | HPLC (C18 column) | ≥98% area |
Residual Solvents | GC-MS | ≤0.1% (v/v) |
Heavy Metals | ICP-MS | ≤10 ppm |
Properties
IUPAC Name |
3-ethyl-2-methylpyridine | |
---|---|---|
Source | PubChem | |
URL | https://pubchem.ncbi.nlm.nih.gov | |
Description | Data deposited in or computed by PubChem | |
InChI |
InChI=1S/C8H11N/c1-3-8-5-4-6-9-7(8)2/h4-6H,3H2,1-2H3 | |
Source | PubChem | |
URL | https://pubchem.ncbi.nlm.nih.gov | |
Description | Data deposited in or computed by PubChem | |
InChI Key |
ZZKDGABMFBCSRP-UHFFFAOYSA-N | |
Source | PubChem | |
URL | https://pubchem.ncbi.nlm.nih.gov | |
Description | Data deposited in or computed by PubChem | |
Canonical SMILES |
CCC1=C(N=CC=C1)C | |
Source | PubChem | |
URL | https://pubchem.ncbi.nlm.nih.gov | |
Description | Data deposited in or computed by PubChem | |
Molecular Formula |
C8H11N | |
Source | PubChem | |
URL | https://pubchem.ncbi.nlm.nih.gov | |
Description | Data deposited in or computed by PubChem | |
DSSTOX Substance ID |
DTXSID0074841 | |
Record name | 3-Ethyl-2-methylpyridine | |
Source | EPA DSSTox | |
URL | https://comptox.epa.gov/dashboard/DTXSID0074841 | |
Description | DSSTox provides a high quality public chemistry resource for supporting improved predictive toxicology. | |
Molecular Weight |
121.18 g/mol | |
Source | PubChem | |
URL | https://pubchem.ncbi.nlm.nih.gov | |
Description | Data deposited in or computed by PubChem | |
CAS No. |
14159-59-2, 27987-10-6 | |
Record name | 3-Ethyl-2-methylpyridine | |
Source | ChemIDplus | |
URL | https://pubchem.ncbi.nlm.nih.gov/substance/?source=chemidplus&sourceid=0014159592 | |
Description | ChemIDplus is a free, web search system that provides access to the structure and nomenclature authority files used for the identification of chemical substances cited in National Library of Medicine (NLM) databases, including the TOXNET system. | |
Record name | Pyridine, ethylmethyl- | |
Source | ChemIDplus | |
URL | https://pubchem.ncbi.nlm.nih.gov/substance/?source=chemidplus&sourceid=0027987106 | |
Description | ChemIDplus is a free, web search system that provides access to the structure and nomenclature authority files used for the identification of chemical substances cited in National Library of Medicine (NLM) databases, including the TOXNET system. | |
Record name | 3-Ethyl-2-methylpyridine | |
Source | EPA DSSTox | |
URL | https://comptox.epa.gov/dashboard/DTXSID0074841 | |
Description | DSSTox provides a high quality public chemistry resource for supporting improved predictive toxicology. | |
Record name | 2-PICOLINE, 3-ETHYL- | |
Source | FDA Global Substance Registration System (GSRS) | |
URL | https://gsrs.ncats.nih.gov/ginas/app/beta/substances/8S6IRA9M3N | |
Description | The FDA Global Substance Registration System (GSRS) enables the efficient and accurate exchange of information on what substances are in regulated products. Instead of relying on names, which vary across regulatory domains, countries, and regions, the GSRS knowledge base makes it possible for substances to be defined by standardized, scientific descriptions. | |
Explanation | Unless otherwise noted, the contents of the FDA website (www.fda.gov), both text and graphics, are not copyrighted. They are in the public domain and may be republished, reprinted and otherwise used freely by anyone without the need to obtain permission from FDA. Credit to the U.S. Food and Drug Administration as the source is appreciated but not required. | |
Synthesis routes and methods
Procedure details
Retrosynthesis Analysis
AI-Powered Synthesis Planning: Our tool employs the Template_relevance Pistachio, Template_relevance Bkms_metabolic, Template_relevance Pistachio_ringbreaker, Template_relevance Reaxys, Template_relevance Reaxys_biocatalysis model, leveraging a vast database of chemical reactions to predict feasible synthetic routes.
One-Step Synthesis Focus: Specifically designed for one-step synthesis, it provides concise and direct routes for your target compounds, streamlining the synthesis process.
Accurate Predictions: Utilizing the extensive PISTACHIO, BKMS_METABOLIC, PISTACHIO_RINGBREAKER, REAXYS, REAXYS_BIOCATALYSIS database, our tool offers high-accuracy predictions, reflecting the latest in chemical research and data.
Strategy Settings
Precursor scoring | Relevance Heuristic |
---|---|
Min. plausibility | 0.01 |
Model | Template_relevance |
Template Set | Pistachio/Bkms_metabolic/Pistachio_ringbreaker/Reaxys/Reaxys_biocatalysis |
Top-N result to add to graph | 6 |
Feasible Synthetic Routes
Disclaimer and Information on In-Vitro Research Products
Please be aware that all articles and product information presented on BenchChem are intended solely for informational purposes. The products available for purchase on BenchChem are specifically designed for in-vitro studies, which are conducted outside of living organisms. In-vitro studies, derived from the Latin term "in glass," involve experiments performed in controlled laboratory settings using cells or tissues. It is important to note that these products are not categorized as medicines or drugs, and they have not received approval from the FDA for the prevention, treatment, or cure of any medical condition, ailment, or disease. We must emphasize that any form of bodily introduction of these products into humans or animals is strictly prohibited by law. It is essential to adhere to these guidelines to ensure compliance with legal and ethical standards in research and experimentation.