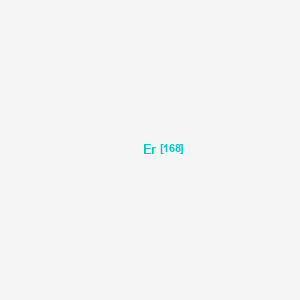
Erbium-168
Overview
Description
Erbium-168 is a stable isotope of the chemical element erbium, which has the atomic number 68. Erbium is a rare earth metal belonging to the lanthanide series of the periodic table. It is a silvery-white metal that is typically found in combination with other elements in minerals such as monazite and xenotime. This compound constitutes approximately 27% of naturally occurring erbium.
Preparation Methods
Synthetic Routes and Reaction Conditions
Erbium-168 can be isolated from its ores through a series of chemical processes. The primary method involves the extraction of erbium from monazite sand, which contains various rare earth elements. The process includes the following steps:
Crushing and Grinding: The ore is crushed and ground to a fine powder.
Acid Leaching: The powdered ore is treated with sulfuric acid to dissolve the rare earth elements.
Solvent Extraction: The solution is subjected to solvent extraction to separate erbium from other rare earth elements.
Precipitation: Erbium is precipitated as erbium hydroxide by adding ammonium hydroxide.
Calcination: The erbium hydroxide is calcined to produce erbium oxide.
Reduction: Erbium oxide is reduced with calcium in a high-temperature furnace to produce metallic erbium.
Industrial Production Methods
Industrial production of this compound involves the use of ion exchange and solvent extraction techniques to purify erbium from its ores. The purified erbium is then subjected to neutron irradiation in a nuclear reactor to produce this compound. This method ensures a high yield of the isotope with minimal impurities.
Chemical Reactions Analysis
Types of Reactions
Erbium-168, like other rare earth elements, primarily undergoes oxidation reactions. It can also participate in reduction and substitution reactions under specific conditions.
Common Reagents and Conditions
Oxidation: this compound reacts with oxygen to form erbium oxide. This reaction occurs readily at elevated temperatures.
Reduction: Erbium oxide can be reduced with calcium to produce metallic erbium.
Substitution: Erbium can form various compounds by substituting other elements in chemical reactions, such as forming erbium chloride by reacting with hydrochloric acid.
Major Products
The major products formed from these reactions include erbium oxide, erbium chloride, and metallic erbium.
Scientific Research Applications
Erbium-168 has several scientific research applications due to its unique properties:
Chemistry: this compound is used as a dopant in various materials to enhance their optical properties. It is commonly used in the production of erbium-doped fiber amplifiers, which are essential components in optical communication systems.
Biology: this compound is used in biological research to study the effects of rare earth elements on biological systems. It is also used in the development of contrast agents for magnetic resonance imaging (MRI).
Medicine: this compound is used in the production of erbium lasers, which are used in various medical procedures, including dermatology and dentistry. These lasers are known for their precision and minimal thermal damage to surrounding tissues.
Industry: this compound is used in the production of specialized glass and ceramics. It is also used in the manufacturing of nuclear reactor control rods due to its neutron absorption properties.
Mechanism of Action
The mechanism by which erbium-168 exerts its effects depends on its application:
Optical Applications: In optical applications, this compound ions absorb and emit light at specific wavelengths, which enhances the performance of optical devices.
Medical Applications: In medical applications, this compound lasers produce precise and controlled thermal effects on tissues, promoting healing and reducing damage to surrounding areas.
Biological Applications: In biological research, this compound interacts with cellular components, allowing researchers to study its effects on cellular processes and structures.
Comparison with Similar Compounds
Erbium-168 can be compared with other rare earth elements in the lanthanide series, such as neodymium, ytterbium, and thulium. Each of these elements has unique properties and applications:
Neodymium: Used in the production of powerful magnets and lasers.
Ytterbium: Used in the production of stainless steel and as a dopant in fiber optics.
Thulium: Used in portable X-ray devices and as a radiation source in cancer treatment.
This compound is unique due to its specific optical properties, making it highly valuable in telecommunications and medical applications.
Properties
IUPAC Name |
erbium-168 | |
---|---|---|
Source | PubChem | |
URL | https://pubchem.ncbi.nlm.nih.gov | |
Description | Data deposited in or computed by PubChem | |
InChI |
InChI=1S/Er/i1+1 | |
Source | PubChem | |
URL | https://pubchem.ncbi.nlm.nih.gov | |
Description | Data deposited in or computed by PubChem | |
InChI Key |
UYAHIZSMUZPPFV-OUBTZVSYSA-N | |
Source | PubChem | |
URL | https://pubchem.ncbi.nlm.nih.gov | |
Description | Data deposited in or computed by PubChem | |
Canonical SMILES |
[Er] | |
Source | PubChem | |
URL | https://pubchem.ncbi.nlm.nih.gov | |
Description | Data deposited in or computed by PubChem | |
Isomeric SMILES |
[168Er] | |
Source | PubChem | |
URL | https://pubchem.ncbi.nlm.nih.gov | |
Description | Data deposited in or computed by PubChem | |
Molecular Formula |
Er | |
Source | PubChem | |
URL | https://pubchem.ncbi.nlm.nih.gov | |
Description | Data deposited in or computed by PubChem | |
DSSTOX Substance ID |
DTXSID30163970 | |
Record name | Erbium, isotope of mass 168 | |
Source | EPA DSSTox | |
URL | https://comptox.epa.gov/dashboard/DTXSID30163970 | |
Description | DSSTox provides a high quality public chemistry resource for supporting improved predictive toxicology. | |
Molecular Weight |
167.932378 g/mol | |
Source | PubChem | |
URL | https://pubchem.ncbi.nlm.nih.gov | |
Description | Data deposited in or computed by PubChem | |
CAS No. |
14833-43-3 | |
Record name | Erbium, isotope of mass 168 | |
Source | ChemIDplus | |
URL | https://pubchem.ncbi.nlm.nih.gov/substance/?source=chemidplus&sourceid=0014833433 | |
Description | ChemIDplus is a free, web search system that provides access to the structure and nomenclature authority files used for the identification of chemical substances cited in National Library of Medicine (NLM) databases, including the TOXNET system. | |
Record name | Erbium, isotope of mass 168 | |
Source | EPA DSSTox | |
URL | https://comptox.epa.gov/dashboard/DTXSID30163970 | |
Description | DSSTox provides a high quality public chemistry resource for supporting improved predictive toxicology. | |
Disclaimer and Information on In-Vitro Research Products
Please be aware that all articles and product information presented on BenchChem are intended solely for informational purposes. The products available for purchase on BenchChem are specifically designed for in-vitro studies, which are conducted outside of living organisms. In-vitro studies, derived from the Latin term "in glass," involve experiments performed in controlled laboratory settings using cells or tissues. It is important to note that these products are not categorized as medicines or drugs, and they have not received approval from the FDA for the prevention, treatment, or cure of any medical condition, ailment, or disease. We must emphasize that any form of bodily introduction of these products into humans or animals is strictly prohibited by law. It is essential to adhere to these guidelines to ensure compliance with legal and ethical standards in research and experimentation.