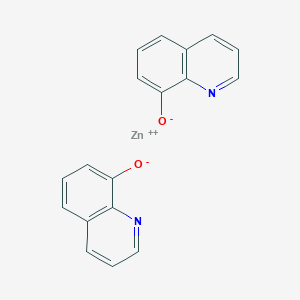
Zinc 8-quinolinolate
Overview
Description
Mechanism of Action
- Zinc 8-quinolinolate primarily targets zinc homeostasis in the body. It plays a crucial role in maintaining intracellular and intra-organellar zinc ion (Zn²⁺) balance .
- This compound interacts with zinc transporters, which facilitate the uptake and release of Zn²⁺ across biological membranes. These transporters maintain zinc homeostasis within cells and organelles .
- This compound impacts several pathways:
- Neurological Disorders : Imbalances in brain zinc are associated with traumatic brain injury, stroke, and seizures. Understanding zinc homeostasis is crucial for preventing and treating these conditions .
- Cellular Effects : Adequate zinc levels are essential for cell growth, immune function, and wound healing. Zinc deficiency leads to stunted growth and impaired wound healing .
Target of Action
Mode of Action
Biochemical Pathways
Result of Action
Biochemical Analysis
Biochemical Properties
Zinc 8-quinolinolate interacts with various biomolecules in the body. As a zinc complex, it plays a role in the regulation of cytokine expression, suppressing inflammation, and activating antioxidant enzymes that scavenge reactive oxygen species . It also participates in lipid, carbohydrate, and protein metabolism .
Cellular Effects
This compound influences various cellular processes. It has been associated with the regulation of chronic inflammatory status through the reduction of inflammatory cytokines . It also reduces oxidative stress by participating in the synthesis of antioxidant enzymes . Furthermore, it acts as a catalyzer of enzymes, taking part in lipid, carbohydrate, and protein metabolism .
Molecular Mechanism
The molecular structure of this compound has been determined by X-ray diffraction . The complex forms triclinic crystals with one [Zn(C9H6NO)2]4 molecule . The molecule is centrosymmetric and tetrameric; four [Zn(C9H6NO)2] units being connected by oxygen atoms of 8-quinolinolate ligands . Two types of bridging oxygen atoms were found . Two crystallographically independent zinc atoms take different geometries, hexa-, and pentacoordinations .
Temporal Effects in Laboratory Settings
The emission spectrum of this compound consists of an intraligand (IL) fluorescence at λmax=550 nm and a rather weak IL phosphorescence at λmax=804 nm . While the appearance of the phosphorescence under ambient conditions is certainly caused by the heavy atom effect of the iodine substituents, their influence is smaller than might be expected .
Dosage Effects in Animal Models
While specific studies on this compound in animal models are limited, research on zinc supplementation has shown that it can improve blood pressure, glucose, and LDL cholesterol serum level . The dosage of Zinc required to produce toxic effects in animals may never be reached in humans .
Metabolic Pathways
Zinc, an essential component of this compound, is involved in numerous metabolic pathways. It is required for the normal function of numerous enzymes, transcriptional factors, and other proteins . These proteins can potentially interact with Zinc through specific regions such as Zinc-finger domains, LIM domains, and RING finger domains .
Transport and Distribution
Zinc transporters regulate Zinc levels by controlling Zinc influx and efflux between extracellular and intracellular compartments, thus, modulating the Zinc concentration and distribution . Zinc transportation is under the control of two major transporter families: SLC30 (ZnT) for the excretion of zinc and SLC39 (ZIP) for the zinc intake .
Subcellular Localization
While specific studies on the subcellular localization of this compound are limited, zinc, an essential component of this compound, is known to be stored in various compartments within the cell . Given the main localization of zinc transporters at the cell membrane, it is likely that this compound follows similar distribution patterns .
Preparation Methods
Synthetic Routes and Reaction Conditions: Zinc 8-quinolinolate can be synthesized through the reaction of zinc salts, such as zinc acetate or zinc chloride, with 8-hydroxyquinoline in an appropriate solvent. The reaction typically involves the following steps:
- Dissolving zinc salt in a solvent like ethanol or methanol.
- Adding 8-hydroxyquinoline to the solution.
- Stirring the mixture at room temperature or slightly elevated temperatures to facilitate the reaction.
- Filtering and washing the precipitated product to obtain pure this compound .
Industrial Production Methods: In industrial settings, the production of this compound may involve similar steps but on a larger scale. The process may include:
- Using large reactors for the reaction.
- Employing continuous stirring and controlled temperature conditions.
- Utilizing filtration and drying equipment to purify and isolate the final product .
Chemical Reactions Analysis
Types of Reactions: Zinc 8-quinolinolate undergoes various chemical reactions, including:
Oxidation: It can be oxidized under specific conditions, leading to the formation of different oxidation states of zinc.
Reduction: Reduction reactions can also occur, altering the oxidation state of zinc within the complex.
Substitution: The ligand (8-hydroxyquinoline) can be substituted with other ligands under certain conditions
Common Reagents and Conditions:
Oxidation: Common oxidizing agents include hydrogen peroxide and oxygen.
Reduction: Reducing agents such as sodium borohydride or lithium aluminum hydride can be used.
Substitution: Ligand exchange reactions can be facilitated by using other ligands like phosphines or amines
Major Products Formed:
Oxidation: Oxidized forms of this compound.
Reduction: Reduced forms of the complex.
Substitution: New complexes with different ligands
Scientific Research Applications
Zinc 8-quinolinolate has a wide range of applications in scientific research:
Comparison with Similar Compounds
Aluminium 8-quinolinolate: Similar in structure but with aluminium as the central metal ion.
Titanium 8-quinolinolate: Contains titanium instead of zinc.
Copper 8-quinolinolate: Copper replaces zinc in the complex
Uniqueness: Zinc 8-quinolinolate is unique due to its specific coordination chemistry and its applications in OLEDs and antimicrobial agents. Its stability and ability to form complexes with various ligands make it distinct from other similar compounds .
Properties
CAS No. |
13978-85-3 |
---|---|
Molecular Formula |
C18H14N2O2Zn |
Molecular Weight |
355.7 g/mol |
IUPAC Name |
quinolin-8-ol;zinc |
InChI |
InChI=1S/2C9H7NO.Zn/c2*11-8-5-1-3-7-4-2-6-10-9(7)8;/h2*1-6,11H; |
InChI Key |
DLJHXMRDIWMMGO-UHFFFAOYSA-N |
SMILES |
C1=CC2=C(C(=C1)[O-])N=CC=C2.C1=CC2=C(C(=C1)[O-])N=CC=C2.[Zn+2] |
Canonical SMILES |
C1=CC2=C(C(=C1)O)N=CC=C2.C1=CC2=C(C(=C1)O)N=CC=C2.[Zn] |
13978-85-3 | |
Pictograms |
Irritant |
Origin of Product |
United States |
Synthesis routes and methods I
Procedure details
Synthesis routes and methods II
Procedure details
Retrosynthesis Analysis
AI-Powered Synthesis Planning: Our tool employs the Template_relevance Pistachio, Template_relevance Bkms_metabolic, Template_relevance Pistachio_ringbreaker, Template_relevance Reaxys, Template_relevance Reaxys_biocatalysis model, leveraging a vast database of chemical reactions to predict feasible synthetic routes.
One-Step Synthesis Focus: Specifically designed for one-step synthesis, it provides concise and direct routes for your target compounds, streamlining the synthesis process.
Accurate Predictions: Utilizing the extensive PISTACHIO, BKMS_METABOLIC, PISTACHIO_RINGBREAKER, REAXYS, REAXYS_BIOCATALYSIS database, our tool offers high-accuracy predictions, reflecting the latest in chemical research and data.
Strategy Settings
Precursor scoring | Relevance Heuristic |
---|---|
Min. plausibility | 0.01 |
Model | Template_relevance |
Template Set | Pistachio/Bkms_metabolic/Pistachio_ringbreaker/Reaxys/Reaxys_biocatalysis |
Top-N result to add to graph | 6 |
Feasible Synthetic Routes
Q1: What is the molecular structure of Zinc 8-quinolinolate and how does this influence its properties?
A1: this compound can exist in both anhydrous and hydrated forms. The anhydrous form, [Zn(C9H6NO)2]4, exhibits a unique tetrameric structure. [] In this structure, four [Zn(C9H6NO)2] units are linked together by oxygen atoms from the 8-quinolinolate ligands, forming a complex with two distinct zinc coordination environments: hexa- and penta-coordination. [] This intricate structure, determined through X-ray diffraction studies, is likely to influence the compound's stability, solubility, and potential applications.
Q2: What are the current methods for synthesizing nanocrystalline this compound?
A2: Recent research highlights two primary methods for synthesizing nanocrystalline this compound:
- Solid-state chemical reaction: This method allows for the production of nanocrystalline this compound at near ambient temperatures. [] This approach offers a potentially more sustainable and energy-efficient alternative to traditional synthesis routes.
- Ultrasonication-assisted solid-state chemical reaction: This method utilizes ultrasound to facilitate the synthesis process. [, ] While further research is needed to fully elucidate the benefits of this method, it may offer advantages in terms of reaction rate and particle size control.
Q3: How is nanocrystalline this compound characterized?
A3: Characterizing the size, morphology, and purity of nanocrystalline this compound is crucial for understanding its properties and potential applications. Researchers employ techniques such as:
- Powder X-ray diffraction (XRD): This technique identifies the crystalline phases present in the sample and provides information about crystallite size. []
- Transmission electron microscopy (TEM): TEM offers direct visualization of the nanoparticles, allowing for the determination of particle size, shape, and distribution. []
Disclaimer and Information on In-Vitro Research Products
Please be aware that all articles and product information presented on BenchChem are intended solely for informational purposes. The products available for purchase on BenchChem are specifically designed for in-vitro studies, which are conducted outside of living organisms. In-vitro studies, derived from the Latin term "in glass," involve experiments performed in controlled laboratory settings using cells or tissues. It is important to note that these products are not categorized as medicines or drugs, and they have not received approval from the FDA for the prevention, treatment, or cure of any medical condition, ailment, or disease. We must emphasize that any form of bodily introduction of these products into humans or animals is strictly prohibited by law. It is essential to adhere to these guidelines to ensure compliance with legal and ethical standards in research and experimentation.