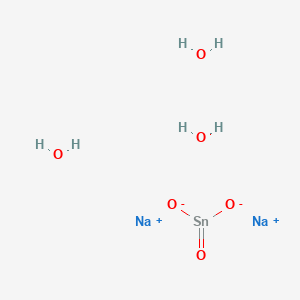
Sodium stannate trihydrate
Overview
Description
Sodium stannate trihydrate, also known as sodium hexahydroxostannate (IV), is an inorganic compound with the chemical formula Na₂[Sn(OH)₆]. This colorless salt forms upon dissolving metallic tin or tin (IV) oxide in sodium hydroxide. It is commonly used as a stabilizer for hydrogen peroxide and has various applications in different fields .
Synthetic Routes and Reaction Conditions:
From Metallic Tin: this compound can be synthesized by dissolving elemental tin in sodium hydroxide solution. The reaction is as follows: [ \text{Sn} + 2\text{NaOH} + 4\text{H₂O} \rightarrow \text{Na₂[Sn(OH)₆]} + 2\text{H₂} ]
From Tin (IV) Oxide: Another method involves dissolving tin (IV) oxide in sodium hydroxide: [ \text{SnO₂} + 2\text{NaOH} + 2\text{H₂O} \rightarrow \text{Na₂[Sn(OH)₆]} ]
Industrial Production Methods:
Roasting with Sodium Carbonate: The anhydrous form of sodium stannate can be prepared by roasting tin (IV) oxide with sodium carbonate in a mixed carbon monoxide and carbon dioxide environment: [ \text{SnO₂} + \text{Na₂CO₃} \rightarrow \text{Na₂SnO₃} + \text{CO₂} ] This method is commonly used in industrial settings.
Types of Reactions:
Oxidation: this compound can undergo oxidation reactions, particularly in the presence of strong oxidizing agents.
Reduction: It can also be reduced to metallic tin under suitable conditions.
Substitution: this compound can participate in substitution reactions where the hydroxyl groups are replaced by other functional groups.
Common Reagents and Conditions:
Oxidizing Agents: Hydrogen peroxide, potassium permanganate.
Reducing Agents: Hydrogen gas, carbon monoxide.
Substitution Reagents: Halogens, acids.
Major Products:
Oxidation: Tin (IV) oxide.
Reduction: Metallic tin.
Substitution: Various tin-based compounds depending on the substituent.
Mechanism of Action
Target of Action
Sodium stannate trihydrate is primarily used as a stabilizer for hydrogen peroxide . It is also used as a precursor to synthesize lanthanum stannate nanoparticles that are used for the modification of glassy carbon electrodes and neodymium stannate nanoparticles for the selective detection of dimetridazole in biological samples .
. .Biochemical Pathways
It is known that the compound plays a role in the synthesis of lanthanum stannate and neodymium stannate nanoparticles . These nanoparticles can be used for various applications, including the modification of glassy carbon electrodes and the selective detection of dimetridazole .
Pharmacokinetics
Given its use as a stabilizer and a precursor in nanoparticle synthesis, it is likely that these properties would depend on the specific application and environment in which the compound is used .
Result of Action
The primary result of this compound’s action is the stabilization of hydrogen peroxide . Additionally, it can be used to synthesize lanthanum stannate and neodymium stannate nanoparticles . These nanoparticles have various applications, such as modifying glassy carbon electrodes and selectively detecting dimetridazole .
Action Environment
The action of this compound can be influenced by various environmental factors. For instance, its ability to stabilize hydrogen peroxide may be affected by the presence of other compounds or changes in temperature . Similarly, the synthesis of nanoparticles using this compound as a precursor can be influenced by factors such as reaction conditions and the presence of other reactants .
Biochemical Analysis
Biochemical Properties
It has been used as a precursor to synthesize lanthanum stannate nanoparticles that are used for the modification of glassy carbon electrodes and neodymium stannate nanoparticles for the selective detection of dimetridazole in biological samples .
Molecular Mechanism
It’s known that the anion is a coordination complex that is octahedral in shape, similar to most stannates .
Scientific Research Applications
Sodium stannate trihydrate has a wide range of applications in scientific research:
Biology: It is employed in the detection of dimetridazole in biological samples.
Comparison with Similar Compounds
Sodium Silicate: Similar in its use as a stabilizer and in industrial applications.
Sodium Germanate: Used in similar applications but with different chemical properties.
Sodium Carbonate: Used in the preparation of sodium stannate trihydrate.
Uniqueness: this compound is unique due to its specific ability to stabilize hydrogen peroxide and its applications in synthesizing specialized nanoparticles for scientific research. Its distinct chemical structure and reactivity make it a valuable compound in various fields .
Properties
IUPAC Name |
disodium;dioxido(oxo)tin;trihydrate | |
---|---|---|
Source | PubChem | |
URL | https://pubchem.ncbi.nlm.nih.gov | |
Description | Data deposited in or computed by PubChem | |
InChI |
InChI=1S/2Na.3H2O.3O.Sn/h;;3*1H2;;;;/q2*+1;;;;;2*-1; | |
Source | PubChem | |
URL | https://pubchem.ncbi.nlm.nih.gov | |
Description | Data deposited in or computed by PubChem | |
InChI Key |
SFXJSNATBHJIDS-UHFFFAOYSA-N | |
Source | PubChem | |
URL | https://pubchem.ncbi.nlm.nih.gov | |
Description | Data deposited in or computed by PubChem | |
Canonical SMILES |
O.O.O.[O-][Sn](=O)[O-].[Na+].[Na+] | |
Source | PubChem | |
URL | https://pubchem.ncbi.nlm.nih.gov | |
Description | Data deposited in or computed by PubChem | |
Molecular Formula |
H6Na2O6Sn | |
Source | PubChem | |
URL | https://pubchem.ncbi.nlm.nih.gov | |
Description | Data deposited in or computed by PubChem | |
Molecular Weight |
266.73 g/mol | |
Source | PubChem | |
URL | https://pubchem.ncbi.nlm.nih.gov | |
Description | Data deposited in or computed by PubChem | |
CAS No. |
12209-98-2 | |
Record name | Sodium stannate | |
Source | ChemIDplus | |
URL | https://pubchem.ncbi.nlm.nih.gov/substance/?source=chemidplus&sourceid=0012209982 | |
Description | ChemIDplus is a free, web search system that provides access to the structure and nomenclature authority files used for the identification of chemical substances cited in National Library of Medicine (NLM) databases, including the TOXNET system. | |
Record name | SODIUM STANNATE | |
Source | FDA Global Substance Registration System (GSRS) | |
URL | https://gsrs.ncats.nih.gov/ginas/app/beta/substances/NJ7C1V83KG | |
Description | The FDA Global Substance Registration System (GSRS) enables the efficient and accurate exchange of information on what substances are in regulated products. Instead of relying on names, which vary across regulatory domains, countries, and regions, the GSRS knowledge base makes it possible for substances to be defined by standardized, scientific descriptions. | |
Explanation | Unless otherwise noted, the contents of the FDA website (www.fda.gov), both text and graphics, are not copyrighted. They are in the public domain and may be republished, reprinted and otherwise used freely by anyone without the need to obtain permission from FDA. Credit to the U.S. Food and Drug Administration as the source is appreciated but not required. | |
Q1: What is sodium stannate trihydrate primarily used for in the presented research?
A1: this compound (Na2SnO3·3H2O) is primarily employed as a catalyst in the ring-opening polymerization (ROP) of ethylene carbonate (EC) in the provided research papers [, , , , , ].
Q2: How does this compound act as a catalyst in this context?
A2: this compound acts as a heterogeneous transesterification catalyst []. It facilitates the opening of the ethylene carbonate ring and the subsequent linking of monomers to form poly(ethylene ether carbonate) diols [].
Q3: What are the structural characteristics of poly(ethylene ether carbonate) diols produced using this compound?
A3: The resulting polymers are not solely composed of carbonate linkages. Due to carbon dioxide emission during polymerization, the polymer chains contain a mixture of carbonate and ether linkages [, ]. The ratio of these linkages can be influenced by factors like temperature, catalyst concentration, and monomer-to-initiator ratio []. The polymers are best described as alternating copolymers of diethylene glycol (DEG) and CO2 [].
Q4: Does the presence of ether linkages impact the properties of the final polymer?
A4: Yes, the presence of ether linkages can impact the polymer's properties. Research indicates that the ratio of carbonate to ether linkages can influence the material's thermal and mechanical properties, impacting its suitability for various applications [].
Q5: How is the structure of these polymers analyzed?
A5: Researchers utilize a combination of techniques to elucidate the complex structure of the poly(ethylene ether carbonate) diols. These include:
- Nuclear Magnetic Resonance (NMR): One-dimensional (1H & 13C) and two-dimensional NMR techniques (COSY, HSQC, HMBC) provide detailed structural information, including the type and arrangement of linkages [, ].
- Capillary Gas Chromatography (CGC): This method, often combined with alkaline degradation of the polymers, allows researchers to analyze the poly(ethylene glycol) components and volatile products, giving insight into the polymer composition [].
- MALDI-TOF MS: This technique helps determine the molecular weight distribution of the polymers, supporting the findings from NMR analyses [].
- Size Exclusion Chromatography: This method helps monitor the molar mass progression of the polymers during synthesis [, ].
Q6: Are there any alternative uses for this compound mentioned in the research?
A6: Yes, this compound, in combination with potassium sodium tartrate tetrahydrate, has been investigated for its synergistic inhibitory effect on the self-corrosion of aluminum in alkaline aluminum-air batteries [].
Q7: What are the implications of this compound's role in aluminum-air battery research?
A7: The research suggests that this compound could contribute to enhancing the lifespan and performance of aluminum-air batteries by mitigating corrosion, a significant challenge in this battery technology [].
Q8: Is there any research on this compound in nanomaterial synthesis?
A8: Yes, one study explores the use of this compound in synthesizing tin dioxide (SnO2) self-assembly nanostructure microspheres []. These microspheres have potential applications in gas-sensitive devices [].
Q9: How does the research on this compound contribute to a more sustainable future?
A9: The research on this compound contributes to a more sustainable future in several ways:
- Developing biodegradable polymers: The synthesis of poly(ethylene ether carbonate) diols using this compound offers a potential route to more sustainable and environmentally friendly polymer materials [, ].
- Improving battery technology: The exploration of this compound in aluminum-air batteries could lead to more efficient and longer-lasting energy storage solutions, which are crucial for the transition to renewable energy sources [].
- Synthesizing nanomaterials with potential in environmental monitoring: The production of SnO2 nanostructure microspheres using this compound opens avenues for creating sensitive gas sensors, which can play a vital role in environmental monitoring and pollution control [].
Disclaimer and Information on In-Vitro Research Products
Please be aware that all articles and product information presented on BenchChem are intended solely for informational purposes. The products available for purchase on BenchChem are specifically designed for in-vitro studies, which are conducted outside of living organisms. In-vitro studies, derived from the Latin term "in glass," involve experiments performed in controlled laboratory settings using cells or tissues. It is important to note that these products are not categorized as medicines or drugs, and they have not received approval from the FDA for the prevention, treatment, or cure of any medical condition, ailment, or disease. We must emphasize that any form of bodily introduction of these products into humans or animals is strictly prohibited by law. It is essential to adhere to these guidelines to ensure compliance with legal and ethical standards in research and experimentation.