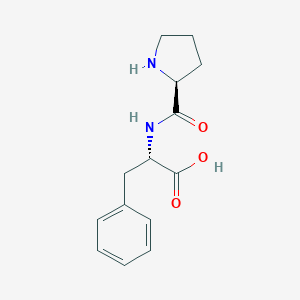
H-Pro-Phe-OH
Overview
Description
H-Pro-Phe-OH is a dipeptide composed of the amino acids proline and phenylalanine. This compound is an incomplete breakdown product of protein digestion or protein catabolism and is found in urine . It exhibits considerable potential for diverse applications in both biochemical and biomedical research .
Mechanism of Action
Target of Action
H-Pro-Phe-OH, also known as Pro-Phe or L-prolyl-L-phenylalanine or L-Pro-L-Phe, is a dipeptide containing proline and phenylalanine . The primary targets of this compound are prolinase and Angiotensin-converting enzyme (ACE) . Prolinase is an enzyme that catalyzes the breakdown of proline-containing peptides, while ACE plays a crucial role in the Renin-Angiotensin System (RAS), which regulates blood pressure .
Mode of Action
This compound serves as a substrate for prolinase . In the context of ACE, phenylalanine, an aromatic amino acid present in the compound, can inhibit the activity of ACE . This inhibition can lead to a decrease in the production of angiotensin II, a potent vasoconstrictor, thereby reducing blood pressure .
Pharmacokinetics
In silico predictions suggest that cyclic peptides, which include this compound, show a better pharmacokinetic and toxic profile to humans .
Result of Action
The inhibition of ACE by this compound leads to a decrease in the production of angiotensin II, resulting in vasodilation and a decrease in blood pressure . This makes this compound potentially useful in the treatment of hypertension.
Biochemical Analysis
Biochemical Properties
L-Prolyl-L-Phenylalanine serves as a substrate for various enzymes, including dipeptidyl peptidase-4 (DPP-4) and prolylcarboxypeptidase (PCP), shedding light on essential biochemical pathways related to protein digestion and metabolism . It interacts with these enzymes, and the nature of these interactions helps us understand the essential biochemical pathways related to protein digestion and metabolism .
Cellular Effects
It is known that it influences cell function, including any impact on cell signaling pathways, gene expression, and cellular metabolism .
Molecular Mechanism
The molecular mechanism of L-Prolyl-L-Phenylalanine is complex. It exerts its effects at the molecular level, including any binding interactions with biomolecules, enzyme inhibition or activation, and changes in gene expression .
Preparation Methods
Synthetic Routes and Reaction Conditions: H-Pro-Phe-OH can be synthesized through various methods. One common approach involves the use of phenylalanine ammonia lyases, which catalyze the interconversion of cinnamic acids and L-phenylalanines . This method is synthetically valuable as it does not require expensive cofactors or recycling systems .
Industrial Production Methods: In industrial settings, recombinant Escherichia coli can be engineered to produce L-phenylalanine, which can then be coupled with proline to form this compound . This method leverages the biosynthesis pathway starting with phosphoenolpyruvate and erythrose 4-phosphate .
Chemical Reactions Analysis
Types of Reactions: H-Pro-Phe-OH undergoes various chemical reactions, including oxidation, reduction, and substitution. It serves as a substrate for enzymes such as dipeptidyl peptidase-4 and prolylcarboxypeptidase .
Common Reagents and Conditions: Common reagents used in these reactions include oxidizing agents for oxidation reactions and reducing agents for reduction reactions. Specific conditions depend on the desired reaction and product.
Major Products: The major products formed from these reactions include various derivatives of this compound, which can be used in further biochemical studies and applications .
Scientific Research Applications
H-Pro-Phe-OH has extensive applications in scientific research:
Chemistry: It is used as a substrate for exploring enzyme active sites and identifying potential drug targets.
Biology: It serves as a tool for studying protein interactions with other molecules.
Industry: It is used in polypeptide synthesis and as a versatile tool in biochemical and metabolomics research.
Comparison with Similar Compounds
- L-azetidine-2-carboxylic acid
- Trans-4-hydroxy-L-proline
- Cis-4-hydroxy-L-proline
Uniqueness: H-Pro-Phe-OH is unique due to its dual composition of proline and phenylalanine, which allows it to serve as a versatile tool in both biochemical and biomedical research . Its ability to inhibit angiotensin-converting enzyme and its potential therapeutic applications further distinguish it from other similar compounds .
Properties
IUPAC Name |
(2S)-3-phenyl-2-[[(2S)-pyrrolidine-2-carbonyl]amino]propanoic acid | |
---|---|---|
Source | PubChem | |
URL | https://pubchem.ncbi.nlm.nih.gov | |
Description | Data deposited in or computed by PubChem | |
InChI |
InChI=1S/C14H18N2O3/c17-13(11-7-4-8-15-11)16-12(14(18)19)9-10-5-2-1-3-6-10/h1-3,5-6,11-12,15H,4,7-9H2,(H,16,17)(H,18,19)/t11-,12-/m0/s1 | |
Source | PubChem | |
URL | https://pubchem.ncbi.nlm.nih.gov | |
Description | Data deposited in or computed by PubChem | |
InChI Key |
IWIANZLCJVYEFX-RYUDHWBXSA-N | |
Source | PubChem | |
URL | https://pubchem.ncbi.nlm.nih.gov | |
Description | Data deposited in or computed by PubChem | |
Canonical SMILES |
C1CC(NC1)C(=O)NC(CC2=CC=CC=C2)C(=O)O | |
Source | PubChem | |
URL | https://pubchem.ncbi.nlm.nih.gov | |
Description | Data deposited in or computed by PubChem | |
Isomeric SMILES |
C1C[C@H](NC1)C(=O)N[C@@H](CC2=CC=CC=C2)C(=O)O | |
Source | PubChem | |
URL | https://pubchem.ncbi.nlm.nih.gov | |
Description | Data deposited in or computed by PubChem | |
Molecular Formula |
C14H18N2O3 | |
Source | PubChem | |
URL | https://pubchem.ncbi.nlm.nih.gov | |
Description | Data deposited in or computed by PubChem | |
Molecular Weight |
262.30 g/mol | |
Source | PubChem | |
URL | https://pubchem.ncbi.nlm.nih.gov | |
Description | Data deposited in or computed by PubChem | |
Physical Description |
Solid | |
Record name | Prolylphenylalanine | |
Source | Human Metabolome Database (HMDB) | |
URL | http://www.hmdb.ca/metabolites/HMDB0011179 | |
Description | The Human Metabolome Database (HMDB) is a freely available electronic database containing detailed information about small molecule metabolites found in the human body. | |
Explanation | HMDB is offered to the public as a freely available resource. Use and re-distribution of the data, in whole or in part, for commercial purposes requires explicit permission of the authors and explicit acknowledgment of the source material (HMDB) and the original publication (see the HMDB citing page). We ask that users who download significant portions of the database cite the HMDB paper in any resulting publications. | |
CAS No. |
13589-02-1 | |
Record name | Prolylphenylalanine | |
Source | Human Metabolome Database (HMDB) | |
URL | http://www.hmdb.ca/metabolites/HMDB0011179 | |
Description | The Human Metabolome Database (HMDB) is a freely available electronic database containing detailed information about small molecule metabolites found in the human body. | |
Explanation | HMDB is offered to the public as a freely available resource. Use and re-distribution of the data, in whole or in part, for commercial purposes requires explicit permission of the authors and explicit acknowledgment of the source material (HMDB) and the original publication (see the HMDB citing page). We ask that users who download significant portions of the database cite the HMDB paper in any resulting publications. | |
Retrosynthesis Analysis
AI-Powered Synthesis Planning: Our tool employs the Template_relevance Pistachio, Template_relevance Bkms_metabolic, Template_relevance Pistachio_ringbreaker, Template_relevance Reaxys, Template_relevance Reaxys_biocatalysis model, leveraging a vast database of chemical reactions to predict feasible synthetic routes.
One-Step Synthesis Focus: Specifically designed for one-step synthesis, it provides concise and direct routes for your target compounds, streamlining the synthesis process.
Accurate Predictions: Utilizing the extensive PISTACHIO, BKMS_METABOLIC, PISTACHIO_RINGBREAKER, REAXYS, REAXYS_BIOCATALYSIS database, our tool offers high-accuracy predictions, reflecting the latest in chemical research and data.
Strategy Settings
Precursor scoring | Relevance Heuristic |
---|---|
Min. plausibility | 0.01 |
Model | Template_relevance |
Template Set | Pistachio/Bkms_metabolic/Pistachio_ringbreaker/Reaxys/Reaxys_biocatalysis |
Top-N result to add to graph | 6 |
Feasible Synthetic Routes
Q1: What is the molecular formula and weight of L-Pro-L-Phe?
A1: L-Pro-L-Phe has a molecular formula of C14H18N2O3 and a molecular weight of 262.31 g/mol.
Q2: Is there any spectroscopic data available for L-Pro-L-Phe?
A2: Yes, NMR spectroscopy has been widely used to elucidate the conformational properties of L-Pro-L-Phe and its derivatives. For instance, researchers used 1H NMR spectroscopy and molecular modeling to compare the conformations of endomorphin-2 (EM2, H-Tyr-Pro-Phe-Phe-NH2) and its C-terminal free acid (EM2OH, H-Tyr-Pro-Phe-Phe-OH) in DMSO solution. []
Q3: Does L-Pro-L-Phe exhibit any catalytic properties?
A3: L-Pro-L-Phe, in combination with p-toluenesulfonic acid (p-TsOH), can act as a catalyst in asymmetric Mannich-type reactions. []
Q4: Can you elaborate on the applications of L-Pro-L-Phe in asymmetric Mannich-type reactions?
A4: Research shows that L-Pro-L-Phe/p-TsOH can efficiently catalyze the direct asymmetric Mannich-type reaction involving acetone, nitrobenzaldehyde, and p-anisidine in DMSO at 0 °C, achieving high enantioselectivity (up to 99% ee). []
Q5: How do structural modifications of L-Pro-L-Phe affect its activity?
A5: Studies on L-Pro-L-Phe analogs in various biological contexts provide insights into SAR:
- Renin Inhibition: Replacing the Leu10-Val11 scissile bond with a phosphinic analog of L-Pro-L-Phe resulted in potent renin inhibitors. []
- Collagenase Inhibition: Cyclizing L-Pro-L-Phe analogs with a phosphinic bond yielded potent inhibitors of bacterial collagenase from Corynebacterium rathaii. The size of the cyclic peptide and stereochemistry of the phenylalanine residue significantly influenced inhibitory potency. []
- Antimicrobial Activity: Cyclo(L-Pro-L-Phe), a cyclic dipeptide derived from Escherichia coli, displayed antimicrobial activity against Ralstonia solanacearum. []
Q6: What are the known biological activities of L-Pro-L-Phe and its derivatives?
A6: Research highlights the following biological activities:
- Neuroprotection: Cyclo(L-Pro-L-Phe) isolated from Aspergillus flavus exhibited neuroprotective effects against oxidative stress-induced neurodegeneration in human neuroblastoma SH-SY5Y cells. It acted as a partial peroxisome proliferator-activated receptor-γ (PPAR-γ) agonist, reducing apoptosis and reactive oxygen species generation. []
- Antimicrobial Activity: Cyclo(L-Pro-L-Phe) showed antimicrobial activity against the plant pathogen Ralstonia solanacearum, significantly inhibiting its growth and reducing disease severity in tomatoes. []
- Angiotensin-Converting Enzyme (ACE) Inhibition: Leu-Pro-Phe, a tripeptide identified from cooked egg digests, displayed ACE inhibitory activity. []
- Cathepsin B Inhibition: The peptide Gly-Pro-Phe-Pro-Ile, corresponding to the sequence 203-207 of bovine β-casein, acted as a competitive inhibitor of cathepsin B. []
Q7: Are there any in vivo studies demonstrating the efficacy of L-Pro-L-Phe or its analogs?
A7: Yes, several studies demonstrate in vivo efficacy:
- Antihypertensive Activity: Oral administration of egg white hydrolysates containing the tripeptide Ile-Val-Phe, structurally related to L-Pro-L-Phe, showed antihypertensive activity in spontaneously hypertensive rats. []
- Alcohol Metabolism: Corn protein hydrolysates containing the peptide Gln-Leu-Leu-Pro-Phe facilitated alcohol metabolism in vivo. The Leu-Leu and Pro-Phe units were identified as key structural features for this activity. []
Q8: What is known about the stability of L-Pro-L-Phe and its analogs?
A8: Research provides insights into the stability of related peptides:
Disclaimer and Information on In-Vitro Research Products
Please be aware that all articles and product information presented on BenchChem are intended solely for informational purposes. The products available for purchase on BenchChem are specifically designed for in-vitro studies, which are conducted outside of living organisms. In-vitro studies, derived from the Latin term "in glass," involve experiments performed in controlled laboratory settings using cells or tissues. It is important to note that these products are not categorized as medicines or drugs, and they have not received approval from the FDA for the prevention, treatment, or cure of any medical condition, ailment, or disease. We must emphasize that any form of bodily introduction of these products into humans or animals is strictly prohibited by law. It is essential to adhere to these guidelines to ensure compliance with legal and ethical standards in research and experimentation.