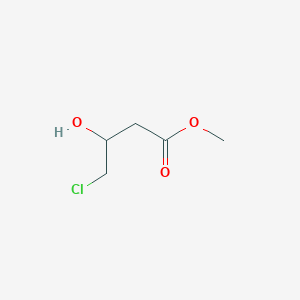
Methyl 4-chloro-3-hydroxybutanoate
Overview
Description
Methyl 4-chloro-3-hydroxybutanoate is an organic compound with the molecular formula C5H9ClO3. It is a chiral molecule, meaning it has non-superimposable mirror images, which is significant in the synthesis of enantiomerically pure compounds. This compound is often used as an intermediate in the synthesis of various pharmaceuticals, particularly those requiring chiral purity.
Mechanism of Action
Target of Action
Methyl 4-chloro-3-hydroxybutanoate is primarily targeted by carbonyl reductase . This enzyme plays a crucial role in the biotransformation of the compound. It is often found in organisms such as Escherichia coli .
Mode of Action
The compound interacts with its target, the carbonyl reductase, through a process known as biotransformation . This interaction results in the conversion of ethyl 4-chloro-3-oxobutanoate (COBE) into ethyl (S)-4-chloro-3-hydroxybutanoate [(S)-CHBE] by the action of the carbonyl reductase .
Biochemical Pathways
The primary biochemical pathway affected by this compound involves the reduction of COBE to (S)-CHBE . This process is catalyzed by the carbonyl reductase enzyme and results in the production of (S)-CHBE, a key chiral intermediate for synthesizing cholesterol-lowering drugs .
Pharmacokinetics
The compound’s bioavailability is likely influenced by its interaction with the carbonyl reductase enzyme and its subsequent biotransformation .
Result of Action
The molecular and cellular effects of this compound’s action primarily involve the production of (S)-CHBE . This compound is a crucial chiral intermediate used in the synthesis of cholesterol-lowering drugs, including hydroxymethylglutaryl-CoA reductase inhibitors .
Action Environment
The action, efficacy, and stability of this compound can be influenced by various environmental factors. For instance, the biotransformation of COBE into (S)-CHBE was found to be more effective when choline chloride/glycerol was used as a biocompatible solvent . Furthermore, the addition of L-glutamine and Tween-80 into the reaction media was shown to improve the biosynthesis of (S)-CHBE .
Preparation Methods
Synthetic Routes and Reaction Conditions: Methyl 4-chloro-3-hydroxybutanoate can be synthesized through the asymmetric reduction of methyl 4-chloro-3-oxobutanoate. This reduction is typically catalyzed by stereoselective enzymes such as carbonyl reductases. The reaction conditions often involve mild temperatures and the presence of a cofactor regeneration system to maintain the activity of the enzyme .
Industrial Production Methods: In industrial settings, the production of this compound often employs biocatalysis due to its high enantioselectivity and efficiency. Recombinant Escherichia coli expressing carbonyl reductase and glucose dehydrogenase genes are commonly used. The process involves culturing the recombinant E. coli in large fermentors, followed by the biocatalytic reduction of methyl 4-chloro-3-oxobutanoate in an aqueous-organic solvent system .
Chemical Reactions Analysis
Types of Reactions: Methyl 4-chloro-3-hydroxybutanoate undergoes various chemical reactions, including:
Oxidation: It can be oxidized to form methyl 4-chloro-3-oxobutanoate.
Reduction: The compound can be reduced to form this compound.
Substitution: The chlorine atom can be substituted with other nucleophiles under appropriate conditions.
Common Reagents and Conditions:
Oxidation: Common oxidizing agents include potassium permanganate and chromium trioxide.
Reduction: Enzymatic reduction using carbonyl reductases is common.
Substitution: Nucleophiles such as sodium azide or thiols can be used for substitution reactions.
Major Products:
Oxidation: Methyl 4-chloro-3-oxobutanoate.
Reduction: this compound.
Substitution: Various substituted derivatives depending on the nucleophile used.
Scientific Research Applications
Methyl 4-chloro-3-hydroxybutanoate is widely used in scientific research due to its role as a chiral intermediate. Its applications include:
Chemistry: Used in the synthesis of chiral compounds and as a building block for complex molecules.
Biology: Employed in studies involving enzyme catalysis and stereoselective reactions.
Medicine: Serves as an intermediate in the production of pharmaceuticals, particularly statins, which are cholesterol-lowering drugs.
Comparison with Similar Compounds
Ethyl 4-chloro-3-hydroxybutanoate: Similar in structure but with an ethyl ester group instead of a methyl ester group.
Methyl 3-hydroxybutanoate: Lacks the chlorine atom, making it less reactive in certain substitution reactions.
Methyl 4-chloro-3-oxobutanoate: The oxidized form of methyl 4-chloro-3-hydroxybutanoate.
Uniqueness: this compound is unique due to its chiral nature and the presence of both a hydroxyl and a chlorine group. This combination allows for a wide range of chemical reactions and makes it a valuable intermediate in the synthesis of enantiomerically pure pharmaceuticals .
Biological Activity
Methyl 4-chloro-3-hydroxybutanoate (MCHB) is a chiral compound with significant biological activity, particularly in the context of enzymatic reactions and pharmaceutical applications. This article explores its biological mechanisms, biochemical properties, and relevant case studies.
Overview of this compound
MCHB is characterized by its chiral nature, which plays a crucial role in its biological efficacy. The compound is primarily synthesized through the asymmetric reduction of ethyl 4-chloro-3-oxobutanoate, often utilizing biocatalytic methods to enhance yield and purity .
Target Enzymes
MCHB primarily interacts with the enzyme carbonyl reductase, which catalyzes the reduction of carbonyl compounds to alcohols. This interaction is vital for the synthesis of various bioactive molecules, including ethyl (R)-2-hydroxy-4-phenylbutyrate, which has therapeutic implications.
Biochemical Pathways
The action of MCHB influences several metabolic pathways. It has been shown to interact with enzymes such as alcohol dehydrogenase from Bartonella apis, affecting cellular metabolism and signaling pathways. This interaction can lead to changes in gene expression and cellular function.
MCHB exhibits several notable biochemical properties:
- Stereochemistry : The (R)-enantiomer of MCHB is often preferred in pharmaceutical applications due to its higher efficacy and selectivity compared to its (S)-enantiomer.
- Solubility : MCHB is soluble in organic solvents, which facilitates its use in various chemical reactions.
- Reactivity : The presence of the hydroxyl and chloro groups enhances its reactivity, making it a valuable intermediate in organic synthesis.
Case Studies and Research Findings
-
Enzymatic Reduction Studies
A study demonstrated that whole cells of Kluyveromyces marxianus were able to bioreduce β-ketoesters, including MCHB, effectively. The conversion rates and enantiomeric excess were analyzed using gas chromatography and infrared spectroscopy . -
Pharmaceutical Applications
MCHB serves as an intermediate in the synthesis of atorvastatin, a widely used cholesterol-lowering drug. Its role in the production process highlights its significance in medicinal chemistry . -
Toxicological Assessments
Research has indicated that MCHB exhibits low toxicity profiles in vitro. These findings are essential for evaluating its safety for use in pharmaceuticals and other applications .
Comparative Analysis
Compound Name | Stereochemistry | Biological Activity | Use Case |
---|---|---|---|
This compound | (R) | Enzyme inhibitor | Intermediate in atorvastatin synthesis |
Ethyl 4-Chloro-3-Hydroxybutanoate | (S) | Lower efficacy | Less preferred for pharmaceutical applications |
Ethyl (R)-2-Hydroxy-4-Phenylbutyrate | (R) | Therapeutic agent | Used in treatments for various metabolic disorders |
Properties
IUPAC Name |
methyl 4-chloro-3-hydroxybutanoate | |
---|---|---|
Source | PubChem | |
URL | https://pubchem.ncbi.nlm.nih.gov | |
Description | Data deposited in or computed by PubChem | |
InChI |
InChI=1S/C5H9ClO3/c1-9-5(8)2-4(7)3-6/h4,7H,2-3H2,1H3 | |
Source | PubChem | |
URL | https://pubchem.ncbi.nlm.nih.gov | |
Description | Data deposited in or computed by PubChem | |
InChI Key |
WMRINGSAVOPXTE-UHFFFAOYSA-N | |
Source | PubChem | |
URL | https://pubchem.ncbi.nlm.nih.gov | |
Description | Data deposited in or computed by PubChem | |
Canonical SMILES |
COC(=O)CC(CCl)O | |
Source | PubChem | |
URL | https://pubchem.ncbi.nlm.nih.gov | |
Description | Data deposited in or computed by PubChem | |
Molecular Formula |
C5H9ClO3 | |
Source | PubChem | |
URL | https://pubchem.ncbi.nlm.nih.gov | |
Description | Data deposited in or computed by PubChem | |
DSSTOX Substance ID |
DTXSID30394469 | |
Record name | methyl 4-chloro-3-hydroxybutanoate | |
Source | EPA DSSTox | |
URL | https://comptox.epa.gov/dashboard/DTXSID30394469 | |
Description | DSSTox provides a high quality public chemistry resource for supporting improved predictive toxicology. | |
Molecular Weight |
152.57 g/mol | |
Source | PubChem | |
URL | https://pubchem.ncbi.nlm.nih.gov | |
Description | Data deposited in or computed by PubChem | |
CAS No. |
10488-68-3 | |
Record name | methyl 4-chloro-3-hydroxybutanoate | |
Source | EPA DSSTox | |
URL | https://comptox.epa.gov/dashboard/DTXSID30394469 | |
Description | DSSTox provides a high quality public chemistry resource for supporting improved predictive toxicology. | |
Retrosynthesis Analysis
AI-Powered Synthesis Planning: Our tool employs the Template_relevance Pistachio, Template_relevance Bkms_metabolic, Template_relevance Pistachio_ringbreaker, Template_relevance Reaxys, Template_relevance Reaxys_biocatalysis model, leveraging a vast database of chemical reactions to predict feasible synthetic routes.
One-Step Synthesis Focus: Specifically designed for one-step synthesis, it provides concise and direct routes for your target compounds, streamlining the synthesis process.
Accurate Predictions: Utilizing the extensive PISTACHIO, BKMS_METABOLIC, PISTACHIO_RINGBREAKER, REAXYS, REAXYS_BIOCATALYSIS database, our tool offers high-accuracy predictions, reflecting the latest in chemical research and data.
Strategy Settings
Precursor scoring | Relevance Heuristic |
---|---|
Min. plausibility | 0.01 |
Model | Template_relevance |
Template Set | Pistachio/Bkms_metabolic/Pistachio_ringbreaker/Reaxys/Reaxys_biocatalysis |
Top-N result to add to graph | 6 |
Feasible Synthetic Routes
Q1: What is the significance of the reaction between methyl 4-chloro-3-hydroxybutanoate and halohydrin dehalogenase?
A1: The reaction between this compound ((R,S)-2) and a mutated halohydrin dehalogenase (Trp249Phe) is significant because it enables the sequential kinetic resolution of the racemic mixture. [] This means the enzyme selectively converts one enantiomer of the substrate ((R)-2) into a different product, leaving the other enantiomer ((S)-2) largely unreacted. This process is highly valuable for obtaining enantiopure compounds, which are important building blocks for pharmaceuticals and other fine chemicals. In this specific case, the reaction yields (S)-methyl 4-cyano-3-hydroxybutanoate ((S)-4) with high enantiomeric excess (96.8% ee), which is a useful building block for statin side-chains. []
Disclaimer and Information on In-Vitro Research Products
Please be aware that all articles and product information presented on BenchChem are intended solely for informational purposes. The products available for purchase on BenchChem are specifically designed for in-vitro studies, which are conducted outside of living organisms. In-vitro studies, derived from the Latin term "in glass," involve experiments performed in controlled laboratory settings using cells or tissues. It is important to note that these products are not categorized as medicines or drugs, and they have not received approval from the FDA for the prevention, treatment, or cure of any medical condition, ailment, or disease. We must emphasize that any form of bodily introduction of these products into humans or animals is strictly prohibited by law. It is essential to adhere to these guidelines to ensure compliance with legal and ethical standards in research and experimentation.