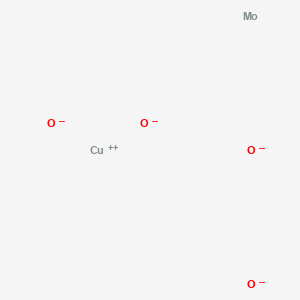
Copper molybdenum tetraoxide
- Click on QUICK INQUIRY to receive a quote from our team of experts.
- With the quality product at a COMPETITIVE price, you can focus more on your research.
Overview
Description
Copper molybdenum tetraoxide, with the chemical formula CuMoO₄, is a compound that combines copper and molybdenum oxides. It is known for its unique properties and potential applications in various fields, including catalysis, electronics, and materials science. The compound is characterized by its high thermal stability, electrical conductivity, and catalytic activity, making it a subject of interest for researchers and industrial applications.
Mechanism of Action
Target of Action
Copper molybdenum tetraoxide, also known as copper molybdate, is a compound that primarily targets the hydrogen evolution reaction (HER) process . This process is crucial for the practical application of hydrogen production, an environmentally friendly and energy-dense fuel .
Mode of Action
The compound interacts with its targets through a process known as doping engineering , which is a strategy to optimize the electrocatalytic activity of electrocatalysts . By incorporating copper atoms into the interlayer of the molybdenum trioxide (MoO3) material, the catalytic activity of the material is optimized . The resulting compound, Cu-MoO3, has a larger surface area, higher conductivity, and strong electron interactions, which contribute to optimal reaction kinetics of the HER process .
Biochemical Pathways
The mechanism of action of molybdenum, a key component of this compound, is complex and involves metabolic interaction with copper, sulfate, and tungsten . Molybdenum is a cofactor for enzymes such as xanthine oxidoreductase, sulfite oxidase, and aldehyde oxidase . These enzymes are involved in various biochemical pathways, affecting a wide range of metabolic processes.
Pharmacokinetics
It’s worth noting that the compound’s high conductivity and large surface area, resulting from the incorporation of copper atoms, may influence its bioavailability .
Result of Action
The primary result of the action of this compound is the optimization of the HER process . The Cu-MoO3 nanosheet only needs a small overpotential of 106 mV to reach the geometric current density of 10 mA cm−2 . This indicates its promising application in hydrogen generation .
Action Environment
The action, efficacy, and stability of this compound can be influenced by various environmental factors. For instance, the compound’s electrocatalytic activity can be optimized through doping engineering, a process that can be affected by the surrounding environment . .
Preparation Methods
Synthetic Routes and Reaction Conditions: Copper molybdenum tetraoxide can be synthesized through various methods, including solid-state reactions, sol-gel processes, and hydrothermal synthesis. One common method involves the solid-state reaction of copper oxide (CuO) and molybdenum trioxide (MoO₃) at high temperatures. The reaction typically occurs at temperatures ranging from 600°C to 800°C, resulting in the formation of CuMoO₄.
Industrial Production Methods: In industrial settings, this compound is often produced through the roasting of copper-containing molybdenite concentrates with sodium salts, followed by water leaching. This process involves the oxidation of molybdenum disulfide (MoS₂) to molybdenum trioxide (MoO₃) and the subsequent reaction with copper compounds to form CuMoO₄. The roasting process is carried out at temperatures between 580°C and 600°C, and the resulting product is purified through hydrometallurgical techniques .
Chemical Reactions Analysis
Types of Reactions: Copper molybdenum tetraoxide undergoes various chemical reactions, including oxidation, reduction, and substitution reactions. These reactions are influenced by the compound’s unique structure and the presence of both copper and molybdenum oxides.
Common Reagents and Conditions:
Oxidation: this compound can be oxidized using strong oxidizing agents such as hydrogen peroxide (H₂O₂) or potassium permanganate (KMnO₄). The oxidation process typically occurs under acidic conditions.
Reduction: The compound can be reduced using reducing agents like hydrogen gas (H₂) or carbon monoxide (CO) at elevated temperatures. The reduction process often leads to the formation of lower oxidation state compounds such as molybdenum dioxide (MoO₂) and copper metal (Cu).
Substitution: Substitution reactions involve the replacement of one or more atoms in the compound with other elements or groups. For example, this compound can react with halogens to form halogenated derivatives.
Major Products Formed: The major products formed from these reactions include molybdenum dioxide (MoO₂), copper metal (Cu), and various halogenated compounds.
Scientific Research Applications
Copper molybdenum tetraoxide has a wide range of scientific research applications due to its unique properties:
Catalysis: The compound is used as a catalyst in various chemical reactions, including hydrogen evolution reactions (HER) and oxygen evolution reactions (OER).
Electronics: this compound is used in the fabrication of electronic devices due to its high electrical conductivity and thermal stability. It is employed in the production of thin films, sensors, and other electronic components.
Materials Science: The compound is studied for its potential use in advanced materials, including nanocomposites and coatings. Its unique structure and properties make it an attractive candidate for developing new materials with enhanced performance.
Environmental Applications: this compound is explored for its potential use in environmental remediation, including the degradation of organic pollutants and the removal of heavy metals from wastewater
Comparison with Similar Compounds
- Molybdenum trioxide (MoO₃)
- Tungsten trioxide (WO₃)
- Molybdenum dioxide (MoO₂)
- Copper oxide (CuO)
- Zinc molybdate (ZnMoO₄)
Properties
CAS No. |
13767-34-5 |
---|---|
Molecular Formula |
CuMoO4 |
Molecular Weight |
223.49 g/mol |
IUPAC Name |
copper;dioxido(dioxo)molybdenum |
InChI |
InChI=1S/Cu.Mo.4O/q+2;;;;2*-1 |
InChI Key |
IKUPISAYGBGQDT-UHFFFAOYSA-N |
SMILES |
[O-2].[O-2].[O-2].[O-2].[Cu+2].[Mo] |
Canonical SMILES |
[O-][Mo](=O)(=O)[O-].[Cu+2] |
Origin of Product |
United States |
Disclaimer and Information on In-Vitro Research Products
Please be aware that all articles and product information presented on BenchChem are intended solely for informational purposes. The products available for purchase on BenchChem are specifically designed for in-vitro studies, which are conducted outside of living organisms. In-vitro studies, derived from the Latin term "in glass," involve experiments performed in controlled laboratory settings using cells or tissues. It is important to note that these products are not categorized as medicines or drugs, and they have not received approval from the FDA for the prevention, treatment, or cure of any medical condition, ailment, or disease. We must emphasize that any form of bodily introduction of these products into humans or animals is strictly prohibited by law. It is essential to adhere to these guidelines to ensure compliance with legal and ethical standards in research and experimentation.