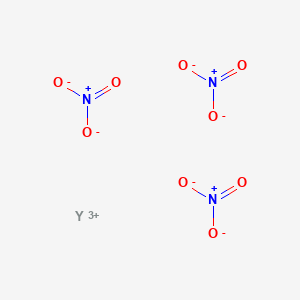
Yttrium nitrate
Overview
Description
Yttrium nitrate is an inorganic compound with the chemical formula Y(NO₃)₃. It is commonly found in its hexahydrate form, Y(NO₃)₃·6H₂O, which appears as colorless crystals. This compound is highly soluble in water and is primarily used as a source of yttrium ions in various chemical processes .
Mechanism of Action
Target of Action
Yttrium nitrate is primarily used as a source of Y^3+ cations . It serves as a precursor for the synthesis of various yttrium-containing materials, such as Y_4Al_2O_9, YBa_2Cu_3O_6.5+x, and yttrium-based metal-organic frameworks . These materials have diverse applications in areas like catalysis, electronics, and materials science .
Mode of Action
The mode of action of this compound involves its interaction with its targets, leading to changes at the molecular level. The thermal decomposition of this compound hexahydrate, Y(NO_3)_3·6H_2O, is a complex condensation process generating a tetramer arrangement Y_4O_4(NO_3)_4 formed by alternating yttrium and oxygen atoms . The anions NO_3^- attach themselves to the yttrium atoms .
Biochemical Pathways
The biochemical pathways affected by this compound are primarily related to its decomposition and the subsequent formation of yttrium oxide. The tetramer gradually loses N_2O_5 and, through the formation of Y_4O_5(NO_3)_2, is transformed into yttrium oxide . This transformation process is crucial in the synthesis of yttrium-containing materials.
Pharmacokinetics
It’s worth noting that a high dose of this compound can induce oxidative stress and cellular apoptosis in the peripheral blood .
Result of Action
The result of this compound’s action is the formation of various yttrium-containing materials. For instance, the addition of this compound hexahydrate improves the dielectric constant and electroactive β phase nucleation in PVDF films . It can also be used as a dopant to prepare cerium oxide hollow sphere hierarchical structures with enhanced photocatalytic activity .
Action Environment
The action of this compound can be influenced by environmental factors such as temperature. For example, the thermal decomposition of this compound hexahydrate begins at relatively low temperatures . Upon further heating, a basic salt YONO_3 is formed . At 600°C, the thermal decomposition is complete, and Y_2O_3 is the final product .
Biochemical Analysis
Biochemical Properties
Yttrium nitrate is soluble in water
Cellular Effects
This compound has been shown to induce oxidative stress and cellular damage in peripheral blood . A high dose of this compound significantly raised the levels of carbonyl protein (CP), monoamine oxidase (MAO), and the cellular apoptosis/necrosis rates of the peripheral blood cells . The total antioxidative capacity (TAOC) and catalase (CAT) levels increased .
Molecular Mechanism
The thermal decomposition of this compound hexahydrate Y(NO3)3·6H2O is a complex condensation process generating a tetramer arrangement Y4O4(NO3)4 formed by alternating yttrium and oxygen atoms . The anions NO3- attach themselves to the yttrium atoms . This process could potentially influence the molecular interactions of this compound in biological systems.
Temporal Effects in Laboratory Settings
This compound hexahydrate loses crystallized water at relatively low temperature . Upon further heating, basic salt YONO3 is formed . At 600°C, the thermal decomposition is complete . These changes over time could potentially affect the long-term effects of this compound on cellular function in laboratory settings.
Dosage Effects in Animal Models
In a study involving pregnant Sprague-Dawley (SD) rats and their offspring, different doses of yttrium (0, 2, 8, and 32 mg/kg.bw) were administered from gestation day 0 to postnatal day 70 . The study found that a dose of 32 mg/kg.bw yttrium significantly raised the levels of CP, MAO, and the cellular apoptosis/necrosis rates of the peripheral blood cells .
Metabolic Pathways
Given its role in inducing oxidative stress , it may interact with metabolic pathways related to oxidative stress response and detoxification.
Preparation Methods
Yttrium nitrate can be synthesized through several methods. One common laboratory method involves dissolving yttrium oxide (Y₂O₃) in nitric acid (HNO₃). The reaction is as follows :
Y2O3+6HNO3→2Y(NO3)3+3H2O
In industrial settings, this compound is often produced by reacting yttrium-containing ores with nitric acid, followed by purification processes to obtain the desired compound.
Chemical Reactions Analysis
Yttrium nitrate undergoes various chemical reactions, including:
-
Thermal Decomposition: : When heated, this compound hexahydrate loses its water of crystallization and decomposes to form yttrium oxide (Y₂O₃) and nitrogen oxides (NOₓ). The decomposition process involves intermediate formation of basic this compound (YONO₃) .
-
Oxidation and Reduction: : this compound can participate in redox reactions, often serving as an oxidizing agent. For example, it can oxidize organic compounds in the presence of a suitable reducing agent.
-
Substitution Reactions: : this compound can react with other anions to form different yttrium salts. For instance, reacting this compound with sodium carbonate (Na₂CO₃) can produce yttrium carbonate (Y₂(CO₃)₃).
Scientific Research Applications
Yttrium nitrate has a wide range of applications in scientific research:
-
Chemistry: : It is used as a precursor for the synthesis of various yttrium-containing materials, such as yttrium aluminum garnet (YAG) and yttrium-based metal-organic frameworks .
-
Biology and Medicine: : this compound is utilized in the preparation of yttrium oxide nanoparticles, which have applications in biological imaging, cancer therapy, and as antibacterial agents .
-
Industry: : In industrial applications, this compound is used in the production of high-performance ceramics, catalysts, and phosphors for light-emitting diodes (LEDs) .
Comparison with Similar Compounds
Yttrium nitrate can be compared with other yttrium compounds such as yttrium chloride (YCl₃), yttrium sulfate (Y₂(SO₄)₃), and yttrium oxide (Y₂O₃). While all these compounds contain yttrium, they differ in their chemical properties and applications:
Yttrium Chloride: Soluble in water and used in the preparation of yttrium-based catalysts and materials.
Yttrium Sulfate: Also soluble in water, used in various chemical synthesis processes.
Yttrium Oxide: Insoluble in water, widely used in ceramics, phosphors, and as a sintering additive.
This compound is unique due to its high solubility in water and its role as a versatile precursor for various yttrium-containing materials.
Properties
CAS No. |
10361-93-0 |
---|---|
Molecular Formula |
HNO3Y |
Molecular Weight |
151.919 g/mol |
IUPAC Name |
nitric acid;yttrium |
InChI |
InChI=1S/HNO3.Y/c2-1(3)4;/h(H,2,3,4); |
InChI Key |
DAHVGXJQRTYNST-UHFFFAOYSA-N |
SMILES |
[N+](=O)([O-])[O-].[N+](=O)([O-])[O-].[N+](=O)([O-])[O-].[Y+3] |
Canonical SMILES |
[N+](=O)(O)[O-].[Y] |
Key on ui other cas no. |
10361-93-0 |
physical_description |
Liquid |
Pictograms |
Oxidizer; Corrosive; Irritant; Environmental Hazard |
Origin of Product |
United States |
Synthesis routes and methods I
Procedure details
Synthesis routes and methods II
Procedure details
Synthesis routes and methods III
Procedure details
Synthesis routes and methods IV
Procedure details
Synthesis routes and methods V
Procedure details
Disclaimer and Information on In-Vitro Research Products
Please be aware that all articles and product information presented on BenchChem are intended solely for informational purposes. The products available for purchase on BenchChem are specifically designed for in-vitro studies, which are conducted outside of living organisms. In-vitro studies, derived from the Latin term "in glass," involve experiments performed in controlled laboratory settings using cells or tissues. It is important to note that these products are not categorized as medicines or drugs, and they have not received approval from the FDA for the prevention, treatment, or cure of any medical condition, ailment, or disease. We must emphasize that any form of bodily introduction of these products into humans or animals is strictly prohibited by law. It is essential to adhere to these guidelines to ensure compliance with legal and ethical standards in research and experimentation.