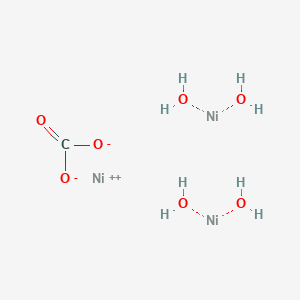
Basic nickel(II) carbonate
- Click on QUICK INQUIRY to receive a quote from our team of experts.
- With the quality product at a COMPETITIVE price, you can focus more on your research.
Overview
Description
Basic nickel(II) carbonate, also known as nickel carbonate hydroxide, is an inorganic compound with the formula Ni₄(CO₃)(OH)₆(H₂O)₄. It is a light green powder that is sparingly soluble in water. This compound is an important intermediate in the hydrometallurgical purification of nickel from its ores and is used in various industrial applications, including electroplating and as a precursor to catalysts .
Scientific Research Applications
Basic nickel(II) carbonate has a wide range of applications in scientific research and industry:
Chemistry: It is used as a precursor to various nickel catalysts and in the synthesis of nickel-based compounds.
Biology: Research on nickel’s role in biological systems often involves this compound as a starting material.
Medicine: While not directly used in medicine, its derivatives and related compounds are studied for their potential therapeutic applications.
Industry: It is used in electroplating, as a pH adjuster, and in the production of ceramic colors and glazes.
Mechanism of Action
Target of Action
Basic nickel(II) carbonate, with the formula Ni4CO3(OH)6(H2O)4 , is a paramagnetic green solid that primarily targets the production of nickel from its ores and is used in electroplating of nickel . It can also serve as a desulfurizer in industrial settings such as natural gas, liquefied petroleum gas, and refineries . Furthermore, it can act as a catalyst in the ethylation reaction .
Mode of Action
The compound interacts with its targets through a series of oxidation and reduction processes . These processes involve a number of elemental reactions and fundamental phenomena, including solid-precursor decomposition, reduction reactions, oxidation reactions, gas- and solid-phase mass transfer, and sintering .
Biochemical Pathways
The biochemical pathways affected by this compound are complex and involve changes in phase, product surface, and internal microstructures . The phenomena affecting the final-product microstructure include chemical changes (i.e., decomposition, reduction reactions, and oxidation reactions), NiO and Ni recrystallization and grain growth, NiO and Ni sintering and densification, and agglomeration of the NiO and Ni particles .
Pharmacokinetics
, which may impact its absorption and distribution. It reacts with acids to produce carbon dioxide , which could influence its metabolism and excretion.
Result of Action
The result of the action of this compound is the production of nickel from its ores . The compound undergoes a series of chemical changes, including decomposition, reduction reactions, and oxidation reactions . These reactions result in changes in the physical and chemical characteristics of the samples, leading to the production of nickel .
Action Environment
The action of this compound is influenced by environmental factors such as temperature, reduction/oxidation time, and partial pressure of hydrogen . For instance, increasing the temperature and reduction time increases the extent of oxygen and sulfur removal . Decreasing the hydrogen partial pressure tenfold lowers the extent of oxygen removal and results in the formation of residual nio (incomplete reduction) . Therefore, it is recommended that the actual process is carried out at temperatures below 600 °C to avoid the formation of this residual NiO .
Safety and Hazards
Nickel(II) Carbonate is classified as Acute Tox. 4 Inhalation - Acute Tox. 4 Oral - Aquatic Acute 1 - Aquatic Chronic 1 - Carc. 1A Inhalation - Muta. 2 - Repr. 1B - Resp. Sens. 1 - Skin Irrit. 2 - Skin Sens. 1 - STOT RE 1 Inhalation . It is recommended to avoid contact with skin, eyes or clothing and use personal protective equipment as required .
Future Directions
A systematic investigation has been carried out to determine the effects of temperature, reduction/oxidation time, and partial pressure of hydrogen on the extent of the oxygen and sulfur removal during basic nickel carbonate (BNC) processing under laboratory conditions . As a result of these studies, it is recommended that the actual process is carried out at temperatures below 600 °C to avoid the formation of this residual NiO .
Biochemical Analysis
Biochemical Properties
The biochemical properties of Basic Nickel(II) Carbonate are primarily observed in its interactions with other compounds during the nickel production process. It undergoes decomposition, reduction reactions, and oxidation reactions . These reactions are complex and involve both gas and solid phases .
Molecular Mechanism
The molecular mechanism of this compound primarily involves its decomposition into nickel oxide and its subsequent reduction to nickel metal . These processes are influenced by factors such as temperature, reduction/oxidation time, and partial pressure of hydrogen .
Temporal Effects in Laboratory Settings
In laboratory settings, the effects of this compound are observed over time during the nickel production process. Increasing the temperature and reduction time increases the extent of oxygen and sulfur removal . Decreasing the hydrogen partial pressure tenfold lowers the extent of oxygen removal and results in the formation of residual nickel oxide .
Metabolic Pathways
The metabolic pathways involving this compound are primarily related to its role in nickel production. The compound undergoes a series of chemical changes, including decomposition, reduction, and oxidation .
Preparation Methods
Synthetic Routes and Reaction Conditions: Basic nickel(II) carbonate can be synthesized by treating solutions of nickel(II) sulfate with sodium carbonate. The reaction typically occurs at room temperature and results in the precipitation of this compound:
NiSO4+Na2CO3+H2O→Ni4(CO3)(OH)6(H2O)4+Na2SO4
Another method involves the electrolytic oxidation of nickel in the presence of carbon dioxide, which forms the hydrated carbonate .
Industrial Production Methods: In industrial settings, this compound is produced through chemical precipitation. Nickel chloride and sodium carbonate are commonly used as raw materials. The process involves washing, drying, re-washing, and drying to achieve high purity . This method is efficient in removing impurities such as sodium and chlorine, which are critical for applications in the electronic industry .
Types of Reactions:
Oxidation: this compound undergoes oxidation to form nickel oxide (NiO) when heated in the presence of air.
Reduction: It can be reduced to metallic nickel (Ni) using hydrogen gas at elevated temperatures.
Hydrolysis: When treated with aqueous acids, this compound hydrolyzes to form nickel(II) ions, water, and carbon dioxide.
Common Reagents and Conditions:
Oxidation: Air or oxygen at temperatures between 110°C and 900°C.
Reduction: Hydrogen gas at temperatures below 600°C to avoid the formation of residual nickel oxide.
Hydrolysis: Aqueous acids such as hydrochloric acid (HCl) at room temperature.
Major Products:
Oxidation: Nickel oxide (NiO)
Reduction: Metallic nickel (Ni)
Hydrolysis: Nickel(II) chloride (NiCl₂), water (H₂O), and carbon dioxide (CO₂).
Comparison with Similar Compounds
Nickel(II) carbonate (NiCO₃): A simpler carbonate that is more commonly encountered in laboratory settings.
Nickel(II) hydroxide (Ni(OH)₂): Used in similar applications but differs in its chemical structure and properties.
Nickel(II) oxide (NiO): A product of the decomposition of basic nickel(II) carbonate and used extensively in catalysis.
Uniqueness: this compound is unique due to its intermediate role in the hydrometallurgical purification of nickel and its specific applications in electroplating and catalyst production. Its ability to undergo multiple types of chemical reactions, including oxidation, reduction, and hydrolysis, makes it a versatile compound in both research and industrial applications .
Properties
{ "Design of the Synthesis Pathway": "The synthesis pathway for Basic nickel(II) carbonate involves the reaction of nickel(II) chloride hexahydrate with sodium carbonate in water, followed by the addition of ammonium hydroxide to precipitate the Basic nickel(II) carbonate.", "Starting Materials": [ "Nickel(II) chloride hexahydrate", "Sodium carbonate", "Water", "Ammonium hydroxide" ], "Reaction": [ "Dissolve 10 g of nickel(II) chloride hexahydrate in 100 mL of water", "Add 5 g of sodium carbonate to the solution and stir until completely dissolved", "Heat the solution to 70°C and maintain for 30 minutes", "Add 10 mL of ammonium hydroxide to the solution and stir for 10 minutes", "Filter the solution to collect the precipitate, Basic nickel(II) carbonate", "Wash the precipitate with water and dry at 60°C for 2 hours" ] } | |
CAS No. |
12607-70-4 |
Molecular Formula |
CH4Ni3O7 |
Molecular Weight |
304.12 g/mol |
IUPAC Name |
nickel(2+);carbonate;tetrahydroxide |
InChI |
InChI=1S/CH2O3.3Ni.4H2O/c2-1(3)4;;;;;;;/h(H2,2,3,4);;;;4*1H2/q;3*+2;;;;/p-6 |
InChI Key |
ATYNVYNPWGEMMI-UHFFFAOYSA-H |
SMILES |
C(=O)([O-])[O-].O.O.O.O.[Ni].[Ni].[Ni+2] |
Canonical SMILES |
C(=O)([O-])[O-].[OH-].[OH-].[OH-].[OH-].[Ni+2].[Ni+2].[Ni+2] |
12607-70-4 | |
Pictograms |
Acute Toxic; Irritant; Health Hazard |
Origin of Product |
United States |
Retrosynthesis Analysis
AI-Powered Synthesis Planning: Our tool employs the Template_relevance Pistachio, Template_relevance Bkms_metabolic, Template_relevance Pistachio_ringbreaker, Template_relevance Reaxys, Template_relevance Reaxys_biocatalysis model, leveraging a vast database of chemical reactions to predict feasible synthetic routes.
One-Step Synthesis Focus: Specifically designed for one-step synthesis, it provides concise and direct routes for your target compounds, streamlining the synthesis process.
Accurate Predictions: Utilizing the extensive PISTACHIO, BKMS_METABOLIC, PISTACHIO_RINGBREAKER, REAXYS, REAXYS_BIOCATALYSIS database, our tool offers high-accuracy predictions, reflecting the latest in chemical research and data.
Strategy Settings
Precursor scoring | Relevance Heuristic |
---|---|
Min. plausibility | 0.01 |
Model | Template_relevance |
Template Set | Pistachio/Bkms_metabolic/Pistachio_ringbreaker/Reaxys/Reaxys_biocatalysis |
Top-N result to add to graph | 6 |
Feasible Synthetic Routes
Q1: What is the significance of studying the magnetic properties of compounds like Ni1−xCuxCr2O4, and what role does basic nickel(II) carbonate play in their synthesis?
A1: Understanding the magnetic properties of mixed metal oxides like Ni1−xCuxCr2O4 is crucial for their potential applications in various fields, including electronics and catalysis. this compound serves as a precursor material in the solid-state synthesis of these compounds. [] Researchers use it alongside other metal carbonates, ensuring a controlled stoichiometry and facilitating the formation of the desired mixed metal oxide at high temperatures. []
Q2: The provided research mentions synthesizing Ni1−xCuxCr2O4 at 800°C. How does the thermal stability of this compound contribute to this synthesis process?
A2: this compound decomposes at high temperatures, releasing carbon dioxide and forming nickel(II) oxide. This thermal decomposition is a crucial step in the solid-state reaction, allowing for the subsequent formation of the desired mixed metal oxide structure. [] The controlled decomposition ensures a homogeneous product with the desired composition.
Q3: Besides solid-state synthesis, are there other applications of this compound in chemical research?
A3: Yes, this compound can also be utilized in molten salt reactions. For instance, research explores its reaction with iron(II) oxalate in a molten lithium carbonate-sodium carbonate-potassium carbonate eutectic. [] This highlights the versatility of this compound as a reagent in different chemical environments, expanding its potential applications in materials science and inorganic synthesis.
Disclaimer and Information on In-Vitro Research Products
Please be aware that all articles and product information presented on BenchChem are intended solely for informational purposes. The products available for purchase on BenchChem are specifically designed for in-vitro studies, which are conducted outside of living organisms. In-vitro studies, derived from the Latin term "in glass," involve experiments performed in controlled laboratory settings using cells or tissues. It is important to note that these products are not categorized as medicines or drugs, and they have not received approval from the FDA for the prevention, treatment, or cure of any medical condition, ailment, or disease. We must emphasize that any form of bodily introduction of these products into humans or animals is strictly prohibited by law. It is essential to adhere to these guidelines to ensure compliance with legal and ethical standards in research and experimentation.