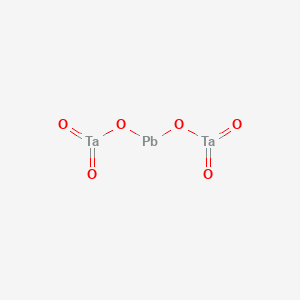
Lead tantalate
Overview
Description
Lead tantalum oxide, with the chemical formula PbTa2O6, is a perovskite material known for its interesting piezoelectric and ferroelectric properties. It has a molecular weight of 665.09 g/mol and is used in various scientific research applications due to its unique characteristics .
Scientific Research Applications
Lead tantalum oxide has a wide range of scientific research applications. Its piezoelectric properties make it suitable for use in sensors, actuators, and energy harvesting devices. It is also used in microelectromechanical systems (MEMS) and ultrasonic transducers.
In addition, lead tantalum oxide exhibits ferroelectric behavior, making it valuable for research in non-volatile memories, such as ferroelectric random-access memory (FRAM).
Mechanism of Action
Target of Action
Lead tantalate, also known as Lead(II) tantalate or Lead tantalum oxide (PbTa2O6), is primarily used in the field of materials science, particularly in the development of electrocaloric materials . These materials are promising working bodies for caloric-based technologies, which are seen as efficient alternatives to vapor compression systems .
Mode of Action
The mode of action of this compound is based on its electrocaloric effect. This effect is a phenomenon where a material shows a change in temperature under an applied electric field . The electrocaloric effect in this compound is triggered by changes in electric, magnetic, or stress field .
Biochemical Pathways
Its primary function is in the realm of materials science and engineering, where it contributes to the operation of devices through its electrocaloric properties .
Result of Action
The result of this compound’s action is a change in temperature when an electric field is applied. This property makes it a promising material for use in electrocaloric devices, such as cooling systems . In fact, it has been shown that a highly ordered bulk lead scandium tantalate can exchange more than a hundred times more electrocaloric heat than the work needed to trigger it .
Action Environment
The action of this compound is influenced by environmental factors such as the strength of the applied electric field. The efficiency of its electrocaloric effect can be optimized by varying the applied electric field . Furthermore, its performance and parameterization can be compromised by net heat transfer between caloric working bodies and heat-transfer fluids .
Safety and Hazards
Future Directions
The new tantalum oxide (Ta2O5)–containing material shows an adequate cytocompatibility and the ability to promote biomineralization without using chemical osteogenic inducers, showing great potential as a new material for vital pulp therapy . Another study shows that Ta2O5 films were synthesized via electron beam evaporation (EBE) and subsequently annealed at different temperatures ranging from 300 to 900 °C .
Preparation Methods
Lead tantalum oxide can be synthesized through several methods. One common approach involves the solid-state reaction of lead oxide (PbO) and tantalum pentoxide (Ta2O5) at high temperatures. The reaction typically occurs in a furnace at temperatures ranging from 800°C to 1000°C. The resulting product is then cooled and ground to obtain the desired compound .
Another method involves the use of sol-gel techniques, where lead and tantalum precursors are dissolved in a solvent, followed by hydrolysis and condensation reactions to form a gel. The gel is then dried and calcined at high temperatures to produce lead tantalum oxide .
Chemical Reactions Analysis
Lead tantalum oxide undergoes various chemical reactions, including oxidation, reduction, and substitution reactions. In oxidation reactions, lead tantalum oxide can react with oxygen to form lead oxide and tantalum pentoxide. In reduction reactions, it can be reduced to its constituent elements, lead and tantalum, under appropriate conditions .
Common reagents used in these reactions include oxygen, hydrogen, and various reducing agents. The major products formed from these reactions depend on the specific conditions and reagents used .
Comparison with Similar Compounds
Lead tantalum oxide can be compared with other similar compounds, such as lead zirconate titanate (PbZrTiO3) and barium strontium titanate (BaSrTiO3). These compounds also exhibit piezoelectric and ferroelectric properties, but lead tantalum oxide is unique due to its specific bandgap and high remnant polarization .
Similar compounds include:
- Lead zirconate titanate (PbZrTiO3)
- Barium strontium titanate (BaSrTiO3)
- Lead scandium tantalate (PbScTaO3)
Lead tantalum oxide stands out due to its unique combination of properties, making it a valuable material for various scientific research applications.
Properties
IUPAC Name |
(dioxotantaliooxy-λ2-plumbanyl)oxy-dioxotantalum | |
---|---|---|
Source | PubChem | |
URL | https://pubchem.ncbi.nlm.nih.gov | |
Description | Data deposited in or computed by PubChem | |
InChI |
InChI=1S/6O.Pb.2Ta | |
Source | PubChem | |
URL | https://pubchem.ncbi.nlm.nih.gov | |
Description | Data deposited in or computed by PubChem | |
InChI Key |
KNSIKTVGKTWOGA-UHFFFAOYSA-N | |
Source | PubChem | |
URL | https://pubchem.ncbi.nlm.nih.gov | |
Description | Data deposited in or computed by PubChem | |
Canonical SMILES |
O=[Ta](=O)O[Pb]O[Ta](=O)=O | |
Source | PubChem | |
URL | https://pubchem.ncbi.nlm.nih.gov | |
Description | Data deposited in or computed by PubChem | |
Molecular Formula |
O6PbTa2 | |
Source | PubChem | |
URL | https://pubchem.ncbi.nlm.nih.gov | |
Description | Data deposited in or computed by PubChem | |
Molecular Weight |
665 g/mol | |
Source | PubChem | |
URL | https://pubchem.ncbi.nlm.nih.gov | |
Description | Data deposited in or computed by PubChem | |
CAS No. |
12065-68-8 | |
Record name | Lead tantalum oxide (PbTa2O6) | |
Source | ChemIDplus | |
URL | https://pubchem.ncbi.nlm.nih.gov/substance/?source=chemidplus&sourceid=0012065688 | |
Description | ChemIDplus is a free, web search system that provides access to the structure and nomenclature authority files used for the identification of chemical substances cited in National Library of Medicine (NLM) databases, including the TOXNET system. | |
Record name | Lead tantalum oxide (PbTa2O6) | |
Source | EPA Chemicals under the TSCA | |
URL | https://www.epa.gov/chemicals-under-tsca | |
Description | EPA Chemicals under the Toxic Substances Control Act (TSCA) collection contains information on chemicals and their regulations under TSCA, including non-confidential content from the TSCA Chemical Substance Inventory and Chemical Data Reporting. | |
Record name | Lead ditantalum hexaoxide | |
Source | European Chemicals Agency (ECHA) | |
URL | https://echa.europa.eu/substance-information/-/substanceinfo/100.031.865 | |
Description | The European Chemicals Agency (ECHA) is an agency of the European Union which is the driving force among regulatory authorities in implementing the EU's groundbreaking chemicals legislation for the benefit of human health and the environment as well as for innovation and competitiveness. | |
Explanation | Use of the information, documents and data from the ECHA website is subject to the terms and conditions of this Legal Notice, and subject to other binding limitations provided for under applicable law, the information, documents and data made available on the ECHA website may be reproduced, distributed and/or used, totally or in part, for non-commercial purposes provided that ECHA is acknowledged as the source: "Source: European Chemicals Agency, http://echa.europa.eu/". Such acknowledgement must be included in each copy of the material. ECHA permits and encourages organisations and individuals to create links to the ECHA website under the following cumulative conditions: Links can only be made to webpages that provide a link to the Legal Notice page. | |
Disclaimer and Information on In-Vitro Research Products
Please be aware that all articles and product information presented on BenchChem are intended solely for informational purposes. The products available for purchase on BenchChem are specifically designed for in-vitro studies, which are conducted outside of living organisms. In-vitro studies, derived from the Latin term "in glass," involve experiments performed in controlled laboratory settings using cells or tissues. It is important to note that these products are not categorized as medicines or drugs, and they have not received approval from the FDA for the prevention, treatment, or cure of any medical condition, ailment, or disease. We must emphasize that any form of bodily introduction of these products into humans or animals is strictly prohibited by law. It is essential to adhere to these guidelines to ensure compliance with legal and ethical standards in research and experimentation.