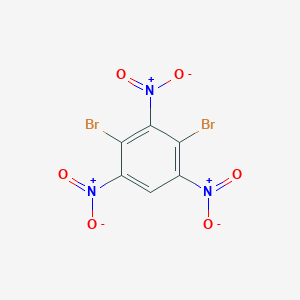
1,3-Dibromo-2,4,6-trinitrobenzene
Overview
Description
1,3-Dibromo-2,4,6-trinitrobenzene is a chemical compound known for its unique structural properties and applications in various fields. It is a two-dimensional charge transfer hyperpolarizable chromophore, which means it has the ability to transfer charge across its structure, making it useful in various optical and electronic applications .
Scientific Research Applications
1,3-Dibromo-2,4,6-trinitrobenzene has several scientific research applications:
Optical Materials: Due to its hyperpolarizable nature, it is used in the development of nonlinear optical materials for applications in photonics and telecommunications.
Electronic Devices: The compound’s charge transfer properties make it useful in the fabrication of electronic devices such as organic semiconductors and sensors.
Chemical Synthesis: It serves as an intermediate in the synthesis of other complex organic molecules, particularly in the field of medicinal chemistry.
Mechanism of Action
Target of Action
1,3-Dibromo-2,4,6-trinitrobenzene (DBTNB) is a two-dimensional charge transfer hyperpolarisable chromophore . It is primarily targeted for its unique properties in the field of crystal engineering and perfect polar alignment .
Mode of Action
DBTNB interacts with its targets by crystallising in the non-centrosymmetric space group C2 in perfect polar order . This interaction leads to an intense powder second harmonic generation (SHG) signal at 1.06 μm .
Biochemical Pathways
It’s known that dbtnb’s mode of action involves the formation of a two-dimensional charge transfer hyperpolarisable chromophore , which could potentially affect various biochemical pathways related to signal transduction.
Result of Action
The primary result of DBTNB’s action is the generation of an intense powder SHG signal at 1.06 μm . This suggests that DBTNB could have potential applications in fields such as optoelectronics and nonlinear optics.
Preparation Methods
Synthetic Routes and Reaction Conditions
1,3-Dibromo-2,4,6-trinitrobenzene can be synthesized through a multi-step process involving the bromination and nitration of benzene derivatives. The typical synthetic route involves the following steps:
Bromination: Benzene is first brominated using bromine in the presence of a catalyst such as iron bromide to form 1,3-dibromobenzene.
Industrial Production Methods
Industrial production of this compound follows similar synthetic routes but on a larger scale. The reaction conditions are optimized to ensure high yield and purity of the final product. The use of continuous flow reactors and advanced separation techniques helps in achieving efficient production.
Chemical Reactions Analysis
1,3-Dibromo-2,4,6-trinitrobenzene undergoes various chemical reactions, including:
Substitution Reactions: The bromine atoms can be substituted by other nucleophiles such as amines or thiols under appropriate conditions.
Reduction Reactions: The nitro groups can be reduced to amino groups using reducing agents like hydrogen gas in the presence of a catalyst.
Oxidation Reactions: The compound can undergo oxidation reactions, although these are less common due to the presence of electron-withdrawing nitro groups.
Common reagents used in these reactions include sodium hydroxide for substitution reactions, hydrogen gas for reduction reactions, and oxidizing agents like potassium permanganate for oxidation reactions. The major products formed depend on the specific reaction conditions and reagents used.
Comparison with Similar Compounds
1,3-Dibromo-2,4,6-trinitrobenzene can be compared with other similar compounds such as:
1,3,5-Triamino-2,4,6-trinitrobenzene: Known for its stability and use in energetic materials.
Hexanitrobenzene: Another highly energetic compound with different applications in explosives and propellants
The uniqueness of this compound lies in its combination of bromine and nitro groups, which imparts distinct charge transfer properties not commonly found in other similar compounds.
Properties
IUPAC Name |
2,4-dibromo-1,3,5-trinitrobenzene | |
---|---|---|
Source | PubChem | |
URL | https://pubchem.ncbi.nlm.nih.gov | |
Description | Data deposited in or computed by PubChem | |
InChI |
InChI=1S/C6HBr2N3O6/c7-4-2(9(12)13)1-3(10(14)15)5(8)6(4)11(16)17/h1H | |
Source | PubChem | |
URL | https://pubchem.ncbi.nlm.nih.gov | |
Description | Data deposited in or computed by PubChem | |
InChI Key |
WTHRTSHMOCVSMH-UHFFFAOYSA-N | |
Source | PubChem | |
URL | https://pubchem.ncbi.nlm.nih.gov | |
Description | Data deposited in or computed by PubChem | |
Canonical SMILES |
C1=C(C(=C(C(=C1[N+](=O)[O-])Br)[N+](=O)[O-])Br)[N+](=O)[O-] | |
Source | PubChem | |
URL | https://pubchem.ncbi.nlm.nih.gov | |
Description | Data deposited in or computed by PubChem | |
Molecular Formula |
C6HBr2N3O6 | |
Source | PubChem | |
URL | https://pubchem.ncbi.nlm.nih.gov | |
Description | Data deposited in or computed by PubChem | |
DSSTOX Substance ID |
DTXSID80407735 | |
Record name | 1,3-DIBROMO-2,4,6-TRINITROBENZENE | |
Source | EPA DSSTox | |
URL | https://comptox.epa.gov/dashboard/DTXSID80407735 | |
Description | DSSTox provides a high quality public chemistry resource for supporting improved predictive toxicology. | |
Molecular Weight |
370.90 g/mol | |
Source | PubChem | |
URL | https://pubchem.ncbi.nlm.nih.gov | |
Description | Data deposited in or computed by PubChem | |
CAS No. |
13506-78-0 | |
Record name | 1,3-DIBROMO-2,4,6-TRINITROBENZENE | |
Source | EPA DSSTox | |
URL | https://comptox.epa.gov/dashboard/DTXSID80407735 | |
Description | DSSTox provides a high quality public chemistry resource for supporting improved predictive toxicology. | |
Retrosynthesis Analysis
AI-Powered Synthesis Planning: Our tool employs the Template_relevance Pistachio, Template_relevance Bkms_metabolic, Template_relevance Pistachio_ringbreaker, Template_relevance Reaxys, Template_relevance Reaxys_biocatalysis model, leveraging a vast database of chemical reactions to predict feasible synthetic routes.
One-Step Synthesis Focus: Specifically designed for one-step synthesis, it provides concise and direct routes for your target compounds, streamlining the synthesis process.
Accurate Predictions: Utilizing the extensive PISTACHIO, BKMS_METABOLIC, PISTACHIO_RINGBREAKER, REAXYS, REAXYS_BIOCATALYSIS database, our tool offers high-accuracy predictions, reflecting the latest in chemical research and data.
Strategy Settings
Precursor scoring | Relevance Heuristic |
---|---|
Min. plausibility | 0.01 |
Model | Template_relevance |
Template Set | Pistachio/Bkms_metabolic/Pistachio_ringbreaker/Reaxys/Reaxys_biocatalysis |
Top-N result to add to graph | 6 |
Feasible Synthetic Routes
Q1: What makes 1,3-Dibromo-2,4,6-trinitrobenzene significant in materials science?
A: this compound exhibits interesting optical properties due to its structure. This compound crystallizes in a non-centrosymmetric pattern with perfect polar alignment of its molecules []. This unique arrangement is highly desirable for non-linear optical applications, as it results in a strong Second Harmonic Generation (SHG) signal at 1.06 microns []. This property makes this compound a potential candidate for use in optical devices and materials.
Q2: How is this compound synthesized?
A: A one-step synthesis method using readily available materials was developed to produce this compound. This method involves reacting meta-dibromobenzene with potassium nitrate in 30% oleum at elevated temperatures (125-130°C) for several hours []. This approach offers a simpler and more efficient alternative to previous multi-step synthesis routes.
Q3: Are there any byproducts formed during the synthesis of this compound, and how are they separated?
A: Yes, a significant byproduct formed during the synthesis is 1,2,3-tribromo-4,6-dinitrobenzene []. This byproduct can be separated from the desired this compound using chromatographic techniques [].
Q4: What are the applications of this compound in chemical synthesis?
A: this compound serves as a valuable precursor in the synthesis of other important compounds. For instance, it can be used to synthesize 2,2',2'',4,4',4'',6,6',6''-nonanitroterphenyl (NONA), a heat-resistant explosive []. This synthesis also yields 2,2',4,4',6,6'-hexanitrobiphenyl (HNB) as a secondary product. Thin-layer chromatography and fractional crystallization techniques are employed to effectively separate and purify these compounds [].
Disclaimer and Information on In-Vitro Research Products
Please be aware that all articles and product information presented on BenchChem are intended solely for informational purposes. The products available for purchase on BenchChem are specifically designed for in-vitro studies, which are conducted outside of living organisms. In-vitro studies, derived from the Latin term "in glass," involve experiments performed in controlled laboratory settings using cells or tissues. It is important to note that these products are not categorized as medicines or drugs, and they have not received approval from the FDA for the prevention, treatment, or cure of any medical condition, ailment, or disease. We must emphasize that any form of bodily introduction of these products into humans or animals is strictly prohibited by law. It is essential to adhere to these guidelines to ensure compliance with legal and ethical standards in research and experimentation.