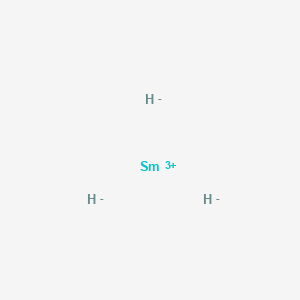
Samarium trihydride
Overview
Description
Samarium is a chemical element with the atomic number 62 and is part of the lanthanide series. It is classified as a rare-earth metal and has a silvery-white appearance. Samarium was discovered in 1879 by French chemist Paul Émile Lecoq de Boisbaudran, who isolated it from the mineral samarskite . This element is relatively stable in air, though it oxidizes slowly over time .
Preparation Methods
Synthetic Routes and Reaction Conditions
Samarium can be prepared through various methods, including the reduction of samarium oxide with lanthanum or cerium. Another common method involves the electrolysis of a molten mixture of samarium chloride and sodium chloride .
Industrial Production Methods
In industrial settings, samarium is often produced by reducing samarium oxide with metallic lanthanum or cerium at high temperatures. The electrolysis method is also employed, where a molten mixture of samarium chloride and sodium chloride is electrolyzed to yield pure samarium .
Chemical Reactions Analysis
Types of Reactions
Samarium undergoes several types of chemical reactions, including:
Oxidation: Samarium reacts with oxygen to form samarium(III) oxide (Sm₂O₃).
Reduction: Samarium(II) iodide (SmI₂) is a powerful reducing agent used in organic synthesis.
Substitution: Samarium reacts with halogens to form samarium halides, such as samarium(III) chloride (SmCl₃) and samarium(III) bromide (SmBr₃).
Common Reagents and Conditions
Oxidation: Reacts with oxygen at room temperature.
Reduction: Samarium(II) iodide is used in tetrahydrofuran (THF) as a solvent.
Substitution: Reacts with halogens like chlorine and bromine at elevated temperatures.
Major Products
Oxidation: Samarium(III) oxide (Sm₂O₃)
Reduction: Various organic compounds reduced by samarium(II) iodide
Substitution: Samarium halides (e.g., SmCl₃, SmBr₃)
Scientific Research Applications
Samarium has a wide range of applications in scientific research:
Chemistry: Used as a catalyst in organic synthesis and in the production of high-performance magnets.
Biology: Samarium complexes are studied for their cytotoxic properties and potential use in cancer treatment.
Medicine: Samarium-153 lexidronam is used in radiopharmaceuticals to treat pain associated with bone cancer.
Industry: Employed in the manufacture of powerful magnets and in the glass and ceramics industry.
Mechanism of Action
Samarium, particularly in its radioactive form (Samarium-153 lexidronam), targets sites of new bone formation. It concentrates in regions of the bone invaded by metastatic tumors, where it irradiates the osteoblastic tumor sites, providing pain relief . The mechanism involves the emission of beta particles that destroy cancer cells in the bone.
Comparison with Similar Compounds
Similar Compounds
Europium: Another lanthanide with similar chemical properties but different applications, such as in phosphorescent materials.
Gadolinium: Used in magnetic resonance imaging (MRI) contrast agents.
Neodymium: Known for its use in strong permanent magnets.
Uniqueness
Samarium is unique due to its specific applications in cancer treatment and its role in producing high-performance magnets. Its ability to form stable complexes with various ligands makes it versatile in both industrial and medical applications .
Biological Activity
Samarium trihydride (SmH) is a compound of samarium, a rare earth element, and hydrogen. Its biological activity has garnered interest due to its potential applications in medicine, particularly in targeted radionuclide therapy and as a therapeutic agent in oncology. This article explores the biological activity of this compound, focusing on its mechanisms of action, therapeutic potential, and relevant case studies.
Overview of this compound
This compound is an inorganic compound that belongs to the class of rare earth hydrides. It is characterized by its ability to release hydrogen gas upon heating, which can be utilized in various chemical reactions. The unique properties of this compound stem from the electronic structure of samarium ions, which can exist in multiple oxidation states (primarily +2 and +3), influencing its reactivity and biological interactions.
Mechanisms of Biological Activity
- Radionuclide Therapy : Samarium-153 (a radioactive isotope of samarium) has been studied extensively for its use in targeted radionuclide therapy (TRNT). When conjugated with targeting agents such as DOTA-TATE, it shows promise in selectively delivering radiation to cancer cells while minimizing damage to surrounding healthy tissues. In vitro studies demonstrated that [Sm]Sm-DOTA-TATE reduced cell viability and clonogenic potential in somatostatin receptor-expressing cancer cells .
- Antimicrobial Activity : Research on samarium complexes indicates that they possess antimicrobial properties. For instance, studies have shown that samarium complexes with fluorinated β-diketones exhibit higher antimicrobial activity compared to their ligands alone . This suggests that the coordination of samarium with specific organic molecules can enhance its biological efficacy.
- Hydride Properties : The hydride form of samarium is known for its nucleophilic characteristics, which may play a role in biochemical reactions involving hydrogen transfer. The nucleophilicity of hydrides can influence their reactivity with various substrates, potentially leading to applications in organic synthesis and medicinal chemistry .
Case Studies
- Targeted Radionuclide Therapy : A study investigated the biodistribution and therapeutic efficacy of [Sm]Sm-DOTA-TATE in mice models with CA20948 tumors. The results indicated a significant uptake of the compound in tumor tissues with rapid clearance from normal tissues, highlighting its potential for clinical applications in treating neuroendocrine tumors .
- Antimicrobial Efficacy : In another study, samarium complexes were tested against various bacterial strains. The results showed that these complexes had a notable inhibitory effect on bacterial growth, suggesting their potential as new antimicrobial agents .
Data Tables
Property | This compound (SmH) | Samarium-153 (Sm-153) |
---|---|---|
Chemical Formula | SmH | Sm-153 |
Oxidation States | +2, +3 | +3 |
Biological Application | Potential antimicrobial agent | Targeted radionuclide therapy |
Mechanism of Action | Nucleophilic hydride transfer | Radiation delivery |
Key Findings | Antimicrobial activity observed | Tumor-selective uptake |
Research Findings
Recent advancements in the production and application of high-molar-activity samarium isotopes have opened new avenues for research. High-molar-activity Sm produced via neutron activation has shown improved stability and efficacy when radiolabeled with targeting agents like DOTA-TATE. This advancement could lead to more effective treatments with reduced side effects for patients undergoing radionuclide therapy .
Properties
IUPAC Name |
samarium | |
---|---|---|
Source | PubChem | |
URL | https://pubchem.ncbi.nlm.nih.gov | |
Description | Data deposited in or computed by PubChem | |
InChI |
InChI=1S/Sm | |
Source | PubChem | |
URL | https://pubchem.ncbi.nlm.nih.gov | |
Description | Data deposited in or computed by PubChem | |
InChI Key |
KZUNJOHGWZRPMI-UHFFFAOYSA-N | |
Source | PubChem | |
URL | https://pubchem.ncbi.nlm.nih.gov | |
Description | Data deposited in or computed by PubChem | |
Canonical SMILES |
[Sm] | |
Source | PubChem | |
URL | https://pubchem.ncbi.nlm.nih.gov | |
Description | Data deposited in or computed by PubChem | |
Molecular Formula |
Sm | |
Source | PubChem | |
URL | https://pubchem.ncbi.nlm.nih.gov | |
Description | Data deposited in or computed by PubChem | |
DSSTOX Substance ID |
DTXSID4064688 | |
Record name | Samarium | |
Source | EPA DSSTox | |
URL | https://comptox.epa.gov/dashboard/DTXSID4064688 | |
Description | DSSTox provides a high quality public chemistry resource for supporting improved predictive toxicology. | |
Molecular Weight |
150.4 g/mol | |
Source | PubChem | |
URL | https://pubchem.ncbi.nlm.nih.gov | |
Description | Data deposited in or computed by PubChem | |
Physical Description |
A hard, silver-white metal [Argonne] Tarnishes on exposure to air; Melting point = 1074 degrees C; [Merck Index] | |
Record name | Samarium | |
Source | Haz-Map, Information on Hazardous Chemicals and Occupational Diseases | |
URL | https://haz-map.com/Agents/1700 | |
Description | Haz-Map® is an occupational health database designed for health and safety professionals and for consumers seeking information about the adverse effects of workplace exposures to chemical and biological agents. | |
Explanation | Copyright (c) 2022 Haz-Map(R). All rights reserved. Unless otherwise indicated, all materials from Haz-Map are copyrighted by Haz-Map(R). No part of these materials, either text or image may be used for any purpose other than for personal use. Therefore, reproduction, modification, storage in a retrieval system or retransmission, in any form or by any means, electronic, mechanical or otherwise, for reasons other than personal use, is strictly prohibited without prior written permission. | |
Boiling Point |
1794 °C | |
Record name | Samarium, Elemental | |
Source | Hazardous Substances Data Bank (HSDB) | |
URL | https://pubchem.ncbi.nlm.nih.gov/source/hsdb/7863 | |
Description | The Hazardous Substances Data Bank (HSDB) is a toxicology database that focuses on the toxicology of potentially hazardous chemicals. It provides information on human exposure, industrial hygiene, emergency handling procedures, environmental fate, regulatory requirements, nanomaterials, and related areas. The information in HSDB has been assessed by a Scientific Review Panel. | |
Density |
7.52 g cu cm at 25 °C | |
Record name | Samarium, Elemental | |
Source | Hazardous Substances Data Bank (HSDB) | |
URL | https://pubchem.ncbi.nlm.nih.gov/source/hsdb/7863 | |
Description | The Hazardous Substances Data Bank (HSDB) is a toxicology database that focuses on the toxicology of potentially hazardous chemicals. It provides information on human exposure, industrial hygiene, emergency handling procedures, environmental fate, regulatory requirements, nanomaterials, and related areas. The information in HSDB has been assessed by a Scientific Review Panel. | |
Vapor Pressure |
1.3X10-5 bar at 722 mm Hg; 1.3X10-3 bar at 964 mm Hg | |
Record name | Samarium, Elemental | |
Source | Hazardous Substances Data Bank (HSDB) | |
URL | https://pubchem.ncbi.nlm.nih.gov/source/hsdb/7863 | |
Description | The Hazardous Substances Data Bank (HSDB) is a toxicology database that focuses on the toxicology of potentially hazardous chemicals. It provides information on human exposure, industrial hygiene, emergency handling procedures, environmental fate, regulatory requirements, nanomaterials, and related areas. The information in HSDB has been assessed by a Scientific Review Panel. | |
Color/Form |
Silvery metal; rhombohedral | |
CAS No. |
13598-53-3, 7440-19-9 | |
Record name | Samarium hydride (SmH3) | |
Source | CAS Common Chemistry | |
URL | https://commonchemistry.cas.org/detail?cas_rn=13598-53-3 | |
Description | CAS Common Chemistry is an open community resource for accessing chemical information. Nearly 500,000 chemical substances from CAS REGISTRY cover areas of community interest, including common and frequently regulated chemicals, and those relevant to high school and undergraduate chemistry classes. This chemical information, curated by our expert scientists, is provided in alignment with our mission as a division of the American Chemical Society. | |
Explanation | The data from CAS Common Chemistry is provided under a CC-BY-NC 4.0 license, unless otherwise stated. | |
Record name | Samarium | |
Source | CAS Common Chemistry | |
URL | https://commonchemistry.cas.org/detail?cas_rn=7440-19-9 | |
Description | CAS Common Chemistry is an open community resource for accessing chemical information. Nearly 500,000 chemical substances from CAS REGISTRY cover areas of community interest, including common and frequently regulated chemicals, and those relevant to high school and undergraduate chemistry classes. This chemical information, curated by our expert scientists, is provided in alignment with our mission as a division of the American Chemical Society. | |
Explanation | The data from CAS Common Chemistry is provided under a CC-BY-NC 4.0 license, unless otherwise stated. | |
Record name | Samarium, elemental | |
Source | ChemIDplus | |
URL | https://pubchem.ncbi.nlm.nih.gov/substance/?source=chemidplus&sourceid=0007440199 | |
Description | ChemIDplus is a free, web search system that provides access to the structure and nomenclature authority files used for the identification of chemical substances cited in National Library of Medicine (NLM) databases, including the TOXNET system. | |
Record name | Samarium | |
Source | DrugBank | |
URL | https://www.drugbank.ca/drugs/DB12403 | |
Description | The DrugBank database is a unique bioinformatics and cheminformatics resource that combines detailed drug (i.e. chemical, pharmacological and pharmaceutical) data with comprehensive drug target (i.e. sequence, structure, and pathway) information. | |
Explanation | Creative Common's Attribution-NonCommercial 4.0 International License (http://creativecommons.org/licenses/by-nc/4.0/legalcode) | |
Record name | Samarium | |
Source | EPA Chemicals under the TSCA | |
URL | https://www.epa.gov/chemicals-under-tsca | |
Description | EPA Chemicals under the Toxic Substances Control Act (TSCA) collection contains information on chemicals and their regulations under TSCA, including non-confidential content from the TSCA Chemical Substance Inventory and Chemical Data Reporting. | |
Record name | Samarium | |
Source | EPA DSSTox | |
URL | https://comptox.epa.gov/dashboard/DTXSID4064688 | |
Description | DSSTox provides a high quality public chemistry resource for supporting improved predictive toxicology. | |
Record name | Samarium | |
Source | European Chemicals Agency (ECHA) | |
URL | https://echa.europa.eu/substance-information/-/substanceinfo/100.028.298 | |
Description | The European Chemicals Agency (ECHA) is an agency of the European Union which is the driving force among regulatory authorities in implementing the EU's groundbreaking chemicals legislation for the benefit of human health and the environment as well as for innovation and competitiveness. | |
Explanation | Use of the information, documents and data from the ECHA website is subject to the terms and conditions of this Legal Notice, and subject to other binding limitations provided for under applicable law, the information, documents and data made available on the ECHA website may be reproduced, distributed and/or used, totally or in part, for non-commercial purposes provided that ECHA is acknowledged as the source: "Source: European Chemicals Agency, http://echa.europa.eu/". Such acknowledgement must be included in each copy of the material. ECHA permits and encourages organisations and individuals to create links to the ECHA website under the following cumulative conditions: Links can only be made to webpages that provide a link to the Legal Notice page. | |
Record name | SAMARIUM | |
Source | FDA Global Substance Registration System (GSRS) | |
URL | https://gsrs.ncats.nih.gov/ginas/app/beta/substances/42OD65L39F | |
Description | The FDA Global Substance Registration System (GSRS) enables the efficient and accurate exchange of information on what substances are in regulated products. Instead of relying on names, which vary across regulatory domains, countries, and regions, the GSRS knowledge base makes it possible for substances to be defined by standardized, scientific descriptions. | |
Explanation | Unless otherwise noted, the contents of the FDA website (www.fda.gov), both text and graphics, are not copyrighted. They are in the public domain and may be republished, reprinted and otherwise used freely by anyone without the need to obtain permission from FDA. Credit to the U.S. Food and Drug Administration as the source is appreciated but not required. | |
Record name | Samarium, Elemental | |
Source | Hazardous Substances Data Bank (HSDB) | |
URL | https://pubchem.ncbi.nlm.nih.gov/source/hsdb/7863 | |
Description | The Hazardous Substances Data Bank (HSDB) is a toxicology database that focuses on the toxicology of potentially hazardous chemicals. It provides information on human exposure, industrial hygiene, emergency handling procedures, environmental fate, regulatory requirements, nanomaterials, and related areas. The information in HSDB has been assessed by a Scientific Review Panel. | |
Record name | 1,2-Diacylglycerol-LD-SM-pool | |
Source | Human Metabolome Database (HMDB) | |
URL | http://www.hmdb.ca/metabolites/HMDB0062272 | |
Description | The Human Metabolome Database (HMDB) is a freely available electronic database containing detailed information about small molecule metabolites found in the human body. | |
Explanation | HMDB is offered to the public as a freely available resource. Use and re-distribution of the data, in whole or in part, for commercial purposes requires explicit permission of the authors and explicit acknowledgment of the source material (HMDB) and the original publication (see the HMDB citing page). We ask that users who download significant portions of the database cite the HMDB paper in any resulting publications. | |
Melting Point |
1072 °C | |
Record name | Samarium, Elemental | |
Source | Hazardous Substances Data Bank (HSDB) | |
URL | https://pubchem.ncbi.nlm.nih.gov/source/hsdb/7863 | |
Description | The Hazardous Substances Data Bank (HSDB) is a toxicology database that focuses on the toxicology of potentially hazardous chemicals. It provides information on human exposure, industrial hygiene, emergency handling procedures, environmental fate, regulatory requirements, nanomaterials, and related areas. The information in HSDB has been assessed by a Scientific Review Panel. | |
Disclaimer and Information on In-Vitro Research Products
Please be aware that all articles and product information presented on BenchChem are intended solely for informational purposes. The products available for purchase on BenchChem are specifically designed for in-vitro studies, which are conducted outside of living organisms. In-vitro studies, derived from the Latin term "in glass," involve experiments performed in controlled laboratory settings using cells or tissues. It is important to note that these products are not categorized as medicines or drugs, and they have not received approval from the FDA for the prevention, treatment, or cure of any medical condition, ailment, or disease. We must emphasize that any form of bodily introduction of these products into humans or animals is strictly prohibited by law. It is essential to adhere to these guidelines to ensure compliance with legal and ethical standards in research and experimentation.