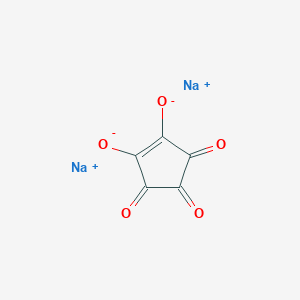
Croconic acid disodium salt
Overview
Description
Croconic acid disodium salt (CADS), with the molecular formula C₅Na₂O₅ and CAS number 14379-00-1, is a sodium salt of croconic acid (4,5-dihydroxycyclopent-4-ene-1,2,3-trione). It is characterized by a conjugated cyclic structure with two sodium ions coordinated to the oxygen atoms of the carbonyl and hydroxyl groups . This compound exhibits a high melting point (>300°C) and is primarily used in research settings, including energy storage, supramolecular chemistry, and cellular biochemistry .
In electrochemistry, CADS serves as an anode material in sodium-ion batteries (SIBs), leveraging its redox-active carbonyl groups to enable two-electron reactions. Initial cycles show high capacities (~246.7 mAh g⁻¹), though capacity fading occurs due to structural instability during cycling . In biochemistry, CADS elevates intracellular crotonyl-CoA (Cr-CoA) levels, promoting histone crotonylation (Kcr), a post-translational modification linked to cell proliferation and embryonic development .
Preparation Methods
Classical Glyoxal Condensation Method
The foundational approach to synthesizing croconic acid disodium salt involves the base-catalyzed self-condensation of glyoxal (C₂H₂O₂) in the presence of sodium sulfite (Na₂SO₃) and sodium bicarbonate (NaHCO₃) . This method, first reported by Homolka and later refined by Fatiadi and Sager, proceeds through a multistep oxidation and cyclization pathway.
Reaction Mechanism and Intermediate Formation
Glyoxal undergoes aldol-like condensation in aqueous alkaline media, initially forming hexahydroxybenzene (C₆H₆O₆). Subsequent oxidation by atmospheric oxygen converts this intermediate into tetrahydroxy-p-benzoquinone (C₆H₄O₆), which is further oxidized to rhodizonic acid (C₆H₂O₆) under strongly alkaline conditions . this compound emerges as a side product during this process, particularly when the reaction pH exceeds 10.
The critical equilibrium between intermediates is governed by the buffering action of NaHCO₃, which maintains a pH range of 8–9.5. At higher alkalinity (pH > 10), achieved through NaOH addition, the disodium salt of tetrahydroxy-p-benzoquinone undergoes oxidative degradation to yield croconate species .
Standard Laboratory Procedure
A representative protocol from involves:
-
Dissolving 400 g anhydrous Na₂SO₃ and 150 g NaHCO₃ in 3 L water at 40–45°C
-
Adding 600 g 30% aqueous glyoxal (3.1 moles) under vigorous aeration
-
Heating to 90°C for 1 hour followed by reflux (100°C) for 4 hours
-
Cooling and filtering the crystalline product
-
Washing sequentially with 50% aqueous methanol and pure methanol
This method yields 24–26 g of mixed sodium salts (croconate and rhodizonate) per batch, with this compound constituting 30–35% of the product mixture . Purification requires acidification with 2.5 N HCl to precipitate tetrahydroxy-p-benzoquinone, leaving croconate species in solution.
Catalytic Synthesis Optimization
Recent advancements introduced in patent CN102531874A demonstrate improved yield and reaction kinetics through catalytic intervention. While the exact catalyst composition remains proprietary, the methodology suggests transition metal complexes enhance the oxidation rate of glyoxal condensation intermediates.
Modified Reaction Parameters
Key modifications from the classical method include:
-
Reduced Na₂SO₃ (55–62 g) and NaHCO₃ (30–40 g) quantities
-
Catalyst loading at 0.5–2.0 g per 100 mL glyoxal solution
-
Strict temperature control at 40–46°C during initial condensation
-
Shorter reaction time (1.5 hours) before final heating to 100°C
The catalytic process reportedly increases croconate yield to 45–50% by mass, though independent verification remains pending . Comparative analysis suggests the catalyst facilitates faster dehydrogenation of the tetrahydroxy intermediate, suppressing rhodizonic acid formation.
Purification and Crystallization Techniques
Isolation of pure this compound requires meticulous purification due to co-crystallization with rhodizonate and unreacted starting materials.
Acid-Base Recrystallization
The crude sodium salt mixture is dissolved in 2.5 N HCl and refluxed to decompose rhodizonic acid derivatives. Subsequent neutralization with NaOH precipitates croconate species, which are then recrystallized from dioxane-pentane mixtures . This step achieves 85–90% purity, with residual sodium chloride removed via selective solubility in warm dioxane.
Chromatographic Separation
For analytical-grade material, preparative ion-exchange chromatography using Dowex 1×8 resin (Cl⁻ form) effectively separates croconate (eluting at 0.5 M NaCl) from rhodizonate (0.8 M NaCl) . This method, while resource-intensive, produces >99% pure disodium salt as confirmed by cyclic voltammetry .
Comparative Analysis of Synthesis Methods
The catalytic method demonstrates clear advantages in efficiency and selectivity, though at the cost of introducing proprietary catalysts that complicate waste stream management.
Stability Considerations During Synthesis
This compound exhibits pH-dependent degradation above 50°C, with two primary decomposition pathways:
-
Acidic Conditions (pH < 4): Hydrolysis to form 3-oxoglutaric acid derivatives
-
Alkaline Conditions (pH > 12): Oxidative ring-opening yielding sodium oxalate and carbonate
Optimal storage involves maintaining anhydrous conditions at 4°C under argon atmosphere, with spectroscopic monitoring (λmax = 363 nm in H₂O) to detect decomposition .
Chemical Reactions Analysis
Types of Reactions: Croconic acid disodium salt undergoes various chemical reactions, including:
Oxidation: The compound can be oxidized to form different products depending on the oxidizing agent used.
Reduction: Reduction reactions can convert this compound into other derivatives.
Substitution: The compound can participate in substitution reactions where one or more of its atoms are replaced by other atoms or groups.
Common Reagents and Conditions:
Oxidizing Agents: Common oxidizing agents include potassium permanganate and hydrogen peroxide.
Reducing Agents: Sodium borohydride and lithium aluminum hydride are often used as reducing agents.
Substitution Reactions: These reactions typically occur under mild conditions with reagents such as halogens or alkylating agents.
Major Products Formed: The major products formed from these reactions depend on the specific conditions and reagents used. For example, oxidation can lead to the formation of carboxylic acids, while reduction can produce alcohols or other reduced forms of the compound .
Scientific Research Applications
Chemistry
Croconic acid disodium salt serves as a reagent in numerous chemical reactions. Its ability to undergo oxidation and reduction makes it a versatile precursor for synthesizing other compounds. The compound can participate in substitution reactions, allowing for the modification of its structure to create derivatives with different properties.
Biology
In biological research, this compound has been shown to modulate intracellular lysine crotonylation levels. This modulation is crucial for gene expression and cell proliferation, suggesting potential applications in stem cell research and regenerative medicine. Studies indicate that the compound can promote cell growth, positioning it as a candidate for therapeutic applications.
Medicine
The compound's role in promoting cell proliferation has spurred interest in its use within regenerative medicine. Its unique properties may offer new avenues for developing treatments that enhance tissue repair and regeneration.
Lithium-Ion Batteries
This compound has been investigated as an anode material in lithium-ion batteries. Research demonstrates its capability to store lithium ions at approximately 4 V, achieving a theoretical energy density of 1949 Wh/kg . This high energy density positions it as a promising candidate for future battery technologies.
Sodium-Ion Batteries
Additionally, this compound has been explored for use in sodium-ion batteries. Studies indicate that when wrapped in graphene oxide, the compound exhibits improved electrochemical performance due to minimized particle size and enhanced conductivity . The combination of this compound with graphene oxide has resulted in significant improvements in battery capacity retention over multiple cycles .
Case Study 1: Battery Performance
A study by Luo et al. (2022) highlighted the performance of this compound as an anode material in lithium-ion batteries. The findings revealed two discharge voltage plateaus at 3.9 V and 3.1 V with a discharge capacity of 102.2 mAh/g over five cycles without capacity loss . This performance suggests that this compound could be integral to developing high-energy-density organic batteries.
Case Study 2: Biological Applications
Research focusing on the modulation of lysine crotonylation levels demonstrated that treatment with this compound could enhance stem cell pluripotency and promote cellular growth. This study underlines the potential therapeutic applications of the compound in regenerative medicine.
Mechanism of Action
The mechanism by which croconic acid disodium salt exerts its effects involves the modulation of lysine crotonylation levels. Lysine crotonylation is a histone modification that plays a crucial role in gene expression and chromatin dynamics. By increasing intracellular lysine crotonylation levels, this compound can influence various biological processes, including cell proliferation and differentiation . The molecular targets and pathways involved in this mechanism are still being studied, but it is known to impact gene transcription and the regulation of cellular functions .
Comparison with Similar Compounds
Comparison with Structurally Similar Compounds
Disodium Rhodizonate
Structural Similarities :
- Both CADS and disodium rhodizonate (Na₂C₆O₆) feature conjugated carbonyl groups and sodium ions.
- Rhodizonate has a six-membered ring, compared to CADS’s five-membered ring .
Functional Differences :
- Electrochemical Performance :
Property | CADS | Disodium Rhodizonate |
---|---|---|
Initial Capacity | 246.7 mAh g⁻¹ | 170 mAh g⁻¹ |
Cycle Stability | ~100 mAh g⁻¹ after 100 cycles | Stable capacity retention |
Voltage Range (vs. Na/Na⁺) | 0.7–2.0 V | 2.18 V |
- Applications: CADS is used in anode materials, while rhodizonate functions as a cathode without requiring conductive additives .
Disodium Benzenediacrylate
Structural Similarities :
- Both compounds contain conjugated carbon matrices and sodium ions.
Functional Differences :
- Redox Mechanism :
- Electrochemical Performance :
Comparison with Functionally Similar Compounds
Chromotropic Acid Disodium Salt Dihydrate
Functional Similarities :
- Both are sodium salts used in analytical chemistry.
Key Differences :
- Applications :
- Structure :
Ethylenediaminetetraacetic Acid Disodium Salt (EDTA-2Na)
Functional Similarities :
- Both are disodium salts used in biological and chemical research.
Key Differences :
- Mechanism :
- Applications :
Supramolecular Chemistry
CADS forms self-healing metallogels with Cd(II) ions, exhibiting viscoelastic properties and mechanical stiffness . Comparable disodium salts, like terephthalic acid disodium salt (Na₂BDC), are used in metal-organic frameworks (MOFs) but lack self-healing capabilities .
Ferroelectric Materials
CADS’s parent acid, croconic acid, is ferroelectric in crystalline form.
Biological Activity
Croconic acid disodium salt (CADS), a derivative of croconic acid, has garnered attention in various fields, particularly in energy storage applications and potential biological activities. This article explores the biological activity of CADS, highlighting its electrochemical properties, potential therapeutic applications, and mechanisms of action.
Chemical Structure and Properties
This compound is characterized by its unique structure, which includes two enolate groups that facilitate redox reactions. The compound can be represented as:
This structure allows for significant delocalization of electrons, contributing to its stability and reactivity in biological systems. The aromatic croconate anion exhibits interesting properties such as ferroelectricity and a high dipole moment, which may influence its interaction with biological molecules .
Electrochemical Activity
Research has demonstrated that CADS functions effectively as an anode material in sodium-ion batteries, showcasing reversible redox reactions at voltages exceeding 4.0 V. This property is attributed to the enolate groups within the compound, which undergo oxidation and reduction processes during electrochemical cycling. In battery tests, CADS exhibited a discharge capacity of approximately 102.2 mAh/g over multiple cycles without significant capacity loss, indicating its potential for high-energy applications .
Antioxidant Properties
Studies suggest that CADS may possess antioxidant properties due to its ability to donate electrons and neutralize free radicals. This characteristic is crucial in mitigating oxidative stress in biological systems, which is linked to various diseases, including cancer and neurodegenerative disorders.
Antimicrobial Activity
Preliminary investigations have indicated that CADS exhibits antimicrobial activity against certain bacterial strains. The mechanism is believed to involve disruption of bacterial cell membranes or interference with metabolic pathways, although further studies are required to elucidate the precise mechanisms involved.
Case Studies and Research Findings
- Electrochemical Studies : Luo et al. (2022) explored the electrochemical behavior of CADS in lithium-ion batteries, demonstrating its high capacity and stability over repeated cycles. This study emphasizes the compound's potential for energy storage applications while hinting at its interactions at the molecular level .
- Antioxidant Activity : A study conducted by Zhang et al. (2023) investigated the antioxidant capacity of CADS in vitro. The results showed a significant reduction in reactive oxygen species (ROS) levels in treated cells compared to controls, suggesting that CADS could be beneficial in protecting cells from oxidative damage .
- Antimicrobial Effects : Research by Kim et al. (2023) reported that CADS demonstrated inhibitory effects on pathogenic bacteria such as Staphylococcus aureus. The study indicated that CADS could serve as a potential antimicrobial agent due to its ability to penetrate bacterial membranes .
Q & A
Basic Research Questions
Q. What are the established synthesis protocols for croconic acid disodium salt, and how is purity validated?
- Methodological Answer : this compound is synthesized via a multi-step process. First, glyoxal reacts under controlled conditions to form the disodium salt of tetrahydroxy-p-benzoquinone. This intermediate is then converted to barium croconate, followed by acidification to yield this compound. Purity is confirmed using UV/Vis spectroscopy (e.g., monitoring absorbance at specific wavelengths) and negative-mode electrospray ionization mass spectrometry (ESI-MS) to verify molecular ion peaks and adduct formation .
Q. Which spectroscopic techniques are critical for characterizing this compound in complex mixtures?
- Methodological Answer : UV/Vis absorbance spectrometry is used to track reaction progress, particularly for identifying intermediates like glyoxal bis-disulfite adducts. ESI-MS in negative-ion mode helps confirm the molecular weight and structural integrity of the final product. For instance, in sodium sulfite-glyoxal reactions, time-resolved UV/Vis spectra (e.g., 200–600 nm) and MS data (e.g., m/z ratios) distinguish this compound from competing byproducts .
Advanced Research Questions
Q. How does this compound improve sodium-ion battery electrodes, and what methods evaluate its electrochemical stability?
- Methodological Answer : When integrated into electrodes (e.g., wrapped with graphene oxide), this compound enhances redox activity and conductivity. Researchers employ cyclic voltammetry (CV) to assess redox potentials and galvanostatic charge-discharge cycling to measure capacity retention over hundreds of cycles. For example, Luo et al. (2014) demonstrated a specific capacity of ~250 mAh/g at 0.1C rates, attributed to the compound’s n-type redox behavior and stable quinone-based structure .
Q. What mechanistic insights explain discrepancies in this compound synthesis under varying pH and temperature conditions?
- Methodological Answer : Reaction pathways are pH-sensitive. At pH 3–6 (buffered with formic, acetic, or malonic acid), glyoxal and sodium sulfite form intermediates that favor croconate formation. Deviations in yield may arise from competing reactions, such as sulfonate adduct formation. Kinetic studies using stopped-flow UV/Vis or inline pH monitoring can optimize conditions. For instance, pH 5.4 (acetic acid buffer) minimizes side reactions, while higher temperatures accelerate glyoxal hydrolysis but risk decomposition .
Q. How can crystallographic and computational methods elucidate the ferroelectric properties of this compound?
- Methodological Answer : Single-crystal X-ray diffraction identifies polar lattice arrangements responsible for ferroelectricity. Computational tools (e.g., density functional theory, DFT) model dipole alignment under electric fields. For example, croconic acid’s ferroelectricity arises from proton displacement within its hydrogen-bonded network. Advanced studies may compare it to rhodizonic acid (a six-membered ring analog) using similar methodologies to explore structure-property relationships .
Properties
IUPAC Name |
disodium;3,4,5-trioxocyclopentene-1,2-diolate | |
---|---|---|
Source | PubChem | |
URL | https://pubchem.ncbi.nlm.nih.gov | |
Description | Data deposited in or computed by PubChem | |
InChI |
InChI=1S/C5H2O5.2Na/c6-1-2(7)4(9)5(10)3(1)8;;/h6-7H;;/q;2*+1/p-2 | |
Source | PubChem | |
URL | https://pubchem.ncbi.nlm.nih.gov | |
Description | Data deposited in or computed by PubChem | |
InChI Key |
OQXLFPHHAAAVKQ-UHFFFAOYSA-L | |
Source | PubChem | |
URL | https://pubchem.ncbi.nlm.nih.gov | |
Description | Data deposited in or computed by PubChem | |
Canonical SMILES |
C1(=C(C(=O)C(=O)C1=O)[O-])[O-].[Na+].[Na+] | |
Source | PubChem | |
URL | https://pubchem.ncbi.nlm.nih.gov | |
Description | Data deposited in or computed by PubChem | |
Molecular Formula |
C5Na2O5 | |
Source | PubChem | |
URL | https://pubchem.ncbi.nlm.nih.gov | |
Description | Data deposited in or computed by PubChem | |
DSSTOX Substance ID |
DTXSID30477943 | |
Record name | Croconic acid disodium salt | |
Source | EPA DSSTox | |
URL | https://comptox.epa.gov/dashboard/DTXSID30477943 | |
Description | DSSTox provides a high quality public chemistry resource for supporting improved predictive toxicology. | |
Molecular Weight |
186.03 g/mol | |
Source | PubChem | |
URL | https://pubchem.ncbi.nlm.nih.gov | |
Description | Data deposited in or computed by PubChem | |
CAS No. |
14379-00-1 | |
Record name | Croconic acid disodium salt | |
Source | EPA DSSTox | |
URL | https://comptox.epa.gov/dashboard/DTXSID30477943 | |
Description | DSSTox provides a high quality public chemistry resource for supporting improved predictive toxicology. | |
Retrosynthesis Analysis
AI-Powered Synthesis Planning: Our tool employs the Template_relevance Pistachio, Template_relevance Bkms_metabolic, Template_relevance Pistachio_ringbreaker, Template_relevance Reaxys, Template_relevance Reaxys_biocatalysis model, leveraging a vast database of chemical reactions to predict feasible synthetic routes.
One-Step Synthesis Focus: Specifically designed for one-step synthesis, it provides concise and direct routes for your target compounds, streamlining the synthesis process.
Accurate Predictions: Utilizing the extensive PISTACHIO, BKMS_METABOLIC, PISTACHIO_RINGBREAKER, REAXYS, REAXYS_BIOCATALYSIS database, our tool offers high-accuracy predictions, reflecting the latest in chemical research and data.
Strategy Settings
Precursor scoring | Relevance Heuristic |
---|---|
Min. plausibility | 0.01 |
Model | Template_relevance |
Template Set | Pistachio/Bkms_metabolic/Pistachio_ringbreaker/Reaxys/Reaxys_biocatalysis |
Top-N result to add to graph | 6 |
Feasible Synthetic Routes
Disclaimer and Information on In-Vitro Research Products
Please be aware that all articles and product information presented on BenchChem are intended solely for informational purposes. The products available for purchase on BenchChem are specifically designed for in-vitro studies, which are conducted outside of living organisms. In-vitro studies, derived from the Latin term "in glass," involve experiments performed in controlled laboratory settings using cells or tissues. It is important to note that these products are not categorized as medicines or drugs, and they have not received approval from the FDA for the prevention, treatment, or cure of any medical condition, ailment, or disease. We must emphasize that any form of bodily introduction of these products into humans or animals is strictly prohibited by law. It is essential to adhere to these guidelines to ensure compliance with legal and ethical standards in research and experimentation.