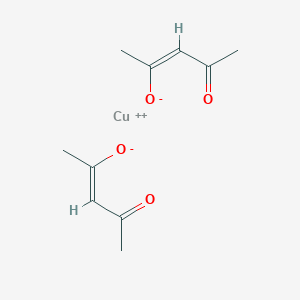
Copper(II) acetylacetonate
Overview
Description
Copper(II) acetylacetonate is a coordination compound with the chemical formula Cu(C₅H₇O₂)₂. It is the homoleptic acetylacetonate complex of copper(II) and exists as a bright blue solid. This compound is insoluble in water and features a square planar geometry around the copper center, as determined by X-ray crystallography .
Preparation Methods
Synthetic Routes and Reaction Conditions: Copper(II) acetylacetonate can be synthesized by reacting copper(II) sulfate with acetylacetone in the presence of a base such as sodium hydroxide. The reaction typically involves mixing an aqueous solution of copper(II) sulfate with acetylacetone and sodium hydroxide, resulting in the formation of a blue precipitate .
Industrial Production Methods: In industrial settings, the preparation of this compound follows similar principles but on a larger scale. The process involves the controlled addition of acetylacetone to a copper(II) salt solution under basic conditions, followed by filtration, washing, and drying of the resulting product .
Chemical Reactions Analysis
Types of Reactions: Copper(II) acetylacetonate participates in various chemical reactions, including oxidation, reduction, and substitution reactions. It is widely used as a catalyst in organic synthesis.
Common Reagents and Conditions:
Oxidation: this compound can catalyze the oxidation of organic substrates using oxidizing agents such as hydrogen peroxide.
Reduction: It can also facilitate the reduction of aromatic nitro compounds to amines.
Substitution: The compound is involved in substitution reactions, such as the aziridination of alkenes using [N-(p-tolylsulfonyl)imino]phenyliodinane as the nitrogen source
Major Products: The major products formed from these reactions depend on the specific substrates and reaction conditions. For example, aziridination of alkenes yields aziridines, while oxidation reactions produce oxidized organic compounds .
Scientific Research Applications
Copper(II) acetylacetonate has numerous applications in scientific research:
Mechanism of Action
The mechanism by which copper(II) acetylacetonate exerts its effects involves the generation of reactive oxygen species and disruption of mitochondrial membrane potential, leading to apoptosis. In catalytic applications, the compound facilitates various organic transformations through coordination with substrates and activation of reaction intermediates .
Comparison with Similar Compounds
- Nickel(II) acetylacetonate
- Cobalt(II) acetylacetonate
- Iron(III) acetylacetonate
Comparison: Copper(II) acetylacetonate is unique due to its specific catalytic properties and applications. While other metal acetylacetonates, such as nickel(II) and cobalt(II) acetylacetonates, also serve as catalysts, this compound is particularly effective in oxidation and aziridination reactions. Additionally, its bright blue color and flexibility in crystal form distinguish it from similar compounds .
Properties
CAS No. |
13395-16-9 |
---|---|
Molecular Formula |
C10H16CuO4 |
Molecular Weight |
263.78 g/mol |
IUPAC Name |
copper;4-hydroxypent-3-en-2-one |
InChI |
InChI=1S/2C5H8O2.Cu/c2*1-4(6)3-5(2)7;/h2*3,6H,1-2H3; |
InChI Key |
ZKXWKVVCCTZOLD-UHFFFAOYSA-N |
Isomeric SMILES |
C/C(=C\C(=O)C)/O.C/C(=C\C(=O)C)/O.[Cu] |
SMILES |
CC(=CC(=O)C)O.CC(=CC(=O)C)O.[Cu] |
Canonical SMILES |
CC(=CC(=O)C)O.CC(=CC(=O)C)O.[Cu] |
boiling_point |
Sublimes |
Color/Form |
Blue powder |
melting_point |
284 C degrees (decomposes) |
13395-16-9 | |
physical_description |
Blue solid; [HSDB] Blue crystalline solid; [MSDSonline] |
Pictograms |
Irritant |
Related CAS |
17272-66-1 (Parent) |
solubility |
Slightly soluble in water; soluble in chloroform |
Synonyms |
acetyl acetonate acetyl acetonate, chromium (III) salt acetyl acetonate, copper (+2) salt acetyl acetonate, manganese (II) salt acetyl acetonate, manganese (III) salt acetyl acetonate, potassium salt acetyl acetonate, sodium salt acetylacetonate chromium(III) acetylacetonate copper acetylacetonate copper(II) acetylacetonate Cu(II) acetyl acetonate cupric acetylacetonate manganese acetylacetonate Nd(III)-acetylacetonate vanadyl acetylacetonate |
Origin of Product |
United States |
Retrosynthesis Analysis
AI-Powered Synthesis Planning: Our tool employs the Template_relevance Pistachio, Template_relevance Bkms_metabolic, Template_relevance Pistachio_ringbreaker, Template_relevance Reaxys, Template_relevance Reaxys_biocatalysis model, leveraging a vast database of chemical reactions to predict feasible synthetic routes.
One-Step Synthesis Focus: Specifically designed for one-step synthesis, it provides concise and direct routes for your target compounds, streamlining the synthesis process.
Accurate Predictions: Utilizing the extensive PISTACHIO, BKMS_METABOLIC, PISTACHIO_RINGBREAKER, REAXYS, REAXYS_BIOCATALYSIS database, our tool offers high-accuracy predictions, reflecting the latest in chemical research and data.
Strategy Settings
Precursor scoring | Relevance Heuristic |
---|---|
Min. plausibility | 0.01 |
Model | Template_relevance |
Template Set | Pistachio/Bkms_metabolic/Pistachio_ringbreaker/Reaxys/Reaxys_biocatalysis |
Top-N result to add to graph | 6 |
Feasible Synthetic Routes
Q1: What is the molecular formula and weight of copper(II) acetylacetonate?
A1: The molecular formula of this compound is Cu(C5H7O2)2 with a molecular weight of 261.75 g/mol. []
Q2: What spectroscopic data is available for characterization?
A2: this compound can be characterized by various techniques. This includes:
- IR spectroscopy: Identifies functional groups and confirms the presence of acetylacetonate ligands. [, , ]
- X-ray diffraction: Reveals the crystal structure and coordination geometry around the copper center, often showing a square planar configuration. []
- EPR spectroscopy: Provides information about the electronic structure and coordination environment of the copper(II) ion. [, , ]
Q3: Is this compound stable in air and moisture?
A3: this compound is generally considered air-stable, but it can be sensitive to moisture. []
Q4: What solvents is this compound soluble in?
A4: It shows good solubility in organic solvents like chloroform, dimethylformamide (DMF), pyridine, and ethanol. [, , , ] The enthalpy change during dissolution in DMF and pyridine indicates specific solvation interactions, particularly with the copper ion. []
Q5: What are the prominent catalytic applications of this compound?
A5: this compound is a versatile catalyst used in various organic reactions:
- Aziridination of styrene: Catalyzes the formation of aziridines from styrene using PhI=NTs as a nitrogen source. While showing higher conversion compared to the homogeneous reaction, diffusion limitations can affect activity. []
- Huisgen-Click Reaction: Efficiently catalyzes the synthesis of 1,2,3-triazoles from organic azides and alkynes in water, suitable for both two- and three-component reactions. []
- Buchner Reaction: Catalyzes the chemospecific intramolecular Buchner reaction of N-benzyl-2-cyano-2-diazoacetamides to synthesize 9-aza-1-cyanobicyclo[5.3.0]deca-2,4,6-trien-10-ones. []
- Oxidative Carbonylation of Methanol: this compound incorporated into Y zeolites exhibits high catalytic activity for the conversion of methanol to dimethyl carbonate, a valuable fuel additive. [, ]
- Aerobic Epoxidation of Styrene: When supported on core-shell structured Fe3O4@SiO2 nanoparticles, it catalyzes styrene epoxidation using air as the oxygen source. This system allows for magnetic recovery and reuse of the catalyst. []
Q6: How does the solvent affect the catalytic activity of this compound?
A6: The choice of solvent significantly influences the catalytic activity of this compound. For instance, in the reduction of aromatic nitro-compounds using sodium borohydride, ethanol proves to be the most effective solvent. [] This emphasizes the importance of solvent optimization in reactions involving this catalyst.
Q7: Are there any examples of this compound being used in heterogeneous catalysis?
A7: Yes, immobilizing this compound on various supports enhances its utility in heterogeneous catalysis. Examples include:
- Amine-functionalized hexagonal mesoporous silica (HMS): Improves styrene conversion in aziridination compared to the homogeneous counterpart, but diffusion limitations are observed. []
- Triamine-functionalized activated carbon: Anchoring via Schiff condensation yields a catalyst active in various reactions. []
- Core-shell structured Fe3O4@SiO2 nanoparticles: Allows magnetic recovery and reuse in styrene epoxidation. []
- Ionic liquids: Facilitates catalyst recycling in aziridination and sulfimidation reactions. [, ]
Q8: Have computational methods been used to study this compound?
A8: Yes, density functional theory (DFT) calculations have been employed to understand solvent effects on the optical and magnetic properties of this compound. These studies successfully modeled solvatochromic shifts in electronic transitions and the influence of solvent basicity on EPR parameters. []
Q9: Can computational methods predict thermoelastic properties of materials containing this compound?
A9: Yes, quasi-harmonic lattice dynamics calculations have been successfully used to determine the thermoelastic moduli of this compound crystals. These calculations reveal anisotropic shrinkage of elastic moduli with increasing temperature, highlighting the importance of considering thermal effects in simulations. []
Q10: How do modifications to the acetylacetonate ligand affect the properties of copper(II) complexes?
A10: Modifications to the acetylacetonate ligand can significantly influence the properties of copper(II) complexes. For example:
- Introduction of oxygen functionalities: Reaction with diacyl peroxides can introduce acyloxy groups into the acetylacetonate ligand, influencing its reactivity. []
Q11: Are there strategies to improve the stability or solubility of this compound?
A11: While this compound is generally stable, its sensitivity to moisture might require specific handling. Encapsulation in a pillared layered clay has been shown to alter its structure and potentially enhance stability. []
Q12: What analytical methods are used to quantify this compound?
A12: Several analytical methods are used for quantification, including:
- Atomic absorption spectroscopy (AAS): Measures the absorption of light by copper atoms to determine the concentration of this compound. [, , ]
- Inductively coupled plasma atomic emission spectroscopy (ICP-AES): A highly sensitive technique for determining the elemental composition of materials, including the copper content in the complex. []
- X-ray photoelectron spectroscopy (XPS): Analyzes the surface composition and chemical states of copper in the complex, providing valuable insights into its interaction with support materials. [, , , , ]
Q13: Are there alternative compounds to this compound in various applications?
A13: Yes, alternative copper precursors have been investigated for applications like atomic layer deposition (ALD), including copper(I)(N,N'-di-sec-butylacetamidinate) and copper(I)(N(1(dimethylvinylsiloxy)-1-methylethano)-2-imino-4-pentanoate) (Cu-KI5). [] The choice of the most suitable precursor depends on the specific application and desired film properties.
Disclaimer and Information on In-Vitro Research Products
Please be aware that all articles and product information presented on BenchChem are intended solely for informational purposes. The products available for purchase on BenchChem are specifically designed for in-vitro studies, which are conducted outside of living organisms. In-vitro studies, derived from the Latin term "in glass," involve experiments performed in controlled laboratory settings using cells or tissues. It is important to note that these products are not categorized as medicines or drugs, and they have not received approval from the FDA for the prevention, treatment, or cure of any medical condition, ailment, or disease. We must emphasize that any form of bodily introduction of these products into humans or animals is strictly prohibited by law. It is essential to adhere to these guidelines to ensure compliance with legal and ethical standards in research and experimentation.