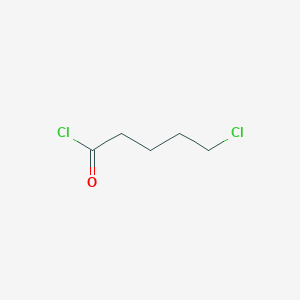
5-Chlorovaleryl chloride
Overview
Description
5-Chlorovaleryl chloride, also known as 5-chloropentanoyl chloride, is an organic compound with the chemical formula C6H11ClO. It is characterized by its chlorine and acyl functional groups, making it a versatile reagent in organic synthesis. This colorless liquid is primarily used in the production of pharmaceuticals, agrochemicals, and dyes .
Scientific Research Applications
5-Chlorovaleryl chloride is widely used in scientific research due to its reactivity and versatility. Some of its applications include:
Safety and Hazards
Future Directions
5-Chlorovaleryl chloride is an important medicine and pesticide intermediate. It is pharmaceutically mainly for the manufacture of the medicine Cilostazole (Cilostazol) for anti-platelet aggregation, protection of vascular endothelial cells, and atherosclerosis . It is also for the production of paddy rice new-type wide-spectrum weedicide pyraclonil (Pyraclonil) .
Mechanism of Action
Target of Action
5-Chlorovaleryl chloride (5-CVC) is primarily used as an alkylating agent in the synthesis of pharmaceutical intermediates and other specialty chemicals . It is a critical component in the production of various active ingredients, including the medicine Cilostazole (Cilostazol) for anti-platelet aggregation and protection of vascular endothelial cells .
Mode of Action
5-CVC interacts with its targets through a process known as alkylation . Alkylation involves the transfer of an alkyl group from the alkylating agent (in this case, 5-CVC) to its target molecule. This process can result in significant changes to the target molecule, altering its function or activity.
Biochemical Pathways
The primary biochemical pathway affected by 5-CVC involves the synthesis of pharmaceutical intermediates and other specialty chemicals . For instance, it has been used in the synthesis of anion-exchange resins by acylation of polystyrene/divinylbenzene and subsequent amination with trimethylamine .
Result of Action
The primary result of 5-CVC’s action is the production of pharmaceutical intermediates and other specialty chemicals . These intermediates can then be further processed to produce a variety of products, including medicines and pesticides .
Action Environment
The action of 5-CVC is highly dependent on the specific environmental conditions in which it is used. For instance, it is sensitive to moisture and should be stored below +30°C . Additionally, it reacts with water, producing hydrochloric acid and 5-chlorovaleric acid . Therefore, careful control of the reaction environment is necessary to ensure the efficacy and stability of 5-CVC.
Biochemical Analysis
Biochemical Properties
It is known to be highly reactive due to the presence of the acyl chloride group, which makes it an excellent reagent in the synthesis of various organic compounds . It has been used in the synthesis of anion-exchange resins by acylation of polystyrene/divinylbenzene and subsequent amination with trimethylamine .
Cellular Effects
It is known to be corrosive and can cause burns of eyes, skin, and mucous membranes . It is also known to be harmful if swallowed .
Molecular Mechanism
The molecular mechanism of 5-Chlorovaleryl chloride involves its reactivity with various biomolecules. It can react by hydrolysis yielding hydrochloric acid and 5-chlorovaleric acid. It can also react with amines yielding amides, with alcohols yielding esters, and with mercaptanes yielding thioesters .
Temporal Effects in Laboratory Settings
It is known to be corrosive and moisture sensitive .
Dosage Effects in Animal Models
It is known to be harmful if swallowed and can cause burns of eyes, skin, and mucous membranes .
Metabolic Pathways
It is known to be highly reactive and can participate in various reactions with biomolecules .
Transport and Distribution
Due to its reactivity, it can potentially interact with various biomolecules and participate in various biochemical reactions .
Subcellular Localization
Due to its reactivity, it can potentially interact with various biomolecules in different subcellular compartments .
Preparation Methods
Synthetic Routes and Reaction Conditions
One common method for synthesizing 5-chlorovaleryl chloride involves the reaction of δ-valerolactone with phosgene in the presence of a catalyst such as iron powder. The reaction is carried out at elevated temperatures (around 100°C) and involves the gradual addition of phosgene over several hours. The reaction mixture is then distilled under reduced pressure to obtain the desired product .
Another method involves the use of 1-bromo-3-chloropropane and diethyl malonate as starting materials. The reaction is carried out in the presence of potassium carbonate powder and a solvent, followed by hydrolysis and subsequent reaction with thionyl chloride to yield this compound .
Industrial Production Methods
Industrial production of this compound typically involves the use of 1,4-dichlorobutane as a raw material. The process includes a three-step reaction: formation of 5-chlorovaleronitrile, hydrolysis to 5-chlorovaleric acid, and finally, conversion to this compound using thionyl chloride .
Chemical Reactions Analysis
Types of Reactions
5-Chlorovaleryl chloride undergoes various types of chemical reactions, including:
Hydrolysis: Reacts with water to yield hydrochloric acid and 5-chlorovaleric acid.
Amination: Reacts with amines to form amides.
Esterification: Reacts with alcohols to form esters.
Thioesterification: Reacts with mercaptans to form thioesters.
Common Reagents and Conditions
Hydrolysis: Water, typically at room temperature.
Amination: Amines, often under mild heating.
Esterification: Alcohols, usually in the presence of a base or acid catalyst.
Thioesterification: Mercaptans, under similar conditions to esterification.
Major Products
Hydrolysis: 5-Chlorovaleric acid and hydrochloric acid.
Amination: Corresponding amides.
Esterification: Corresponding esters.
Thioesterification: Corresponding thioesters.
Comparison with Similar Compounds
5-Chlorovaleryl chloride can be compared with other similar compounds such as:
Valeryl chloride: Lacks the chlorine atom, making it less reactive in certain substitution reactions.
2-Chloropropionyl chloride: Contains a shorter carbon chain and different reactivity profile.
Pivaloyl chloride: Contains a tert-butyl group instead of a linear chain, leading to different steric and electronic effects.
These comparisons highlight the unique reactivity and applications of this compound, particularly in the synthesis of pharmaceuticals and agrochemicals.
Properties
IUPAC Name |
5-chloropentanoyl chloride | |
---|---|---|
Source | PubChem | |
URL | https://pubchem.ncbi.nlm.nih.gov | |
Description | Data deposited in or computed by PubChem | |
InChI |
InChI=1S/C5H8Cl2O/c6-4-2-1-3-5(7)8/h1-4H2 | |
Source | PubChem | |
URL | https://pubchem.ncbi.nlm.nih.gov | |
Description | Data deposited in or computed by PubChem | |
InChI Key |
SVNNWKWHLOJLOK-UHFFFAOYSA-N | |
Source | PubChem | |
URL | https://pubchem.ncbi.nlm.nih.gov | |
Description | Data deposited in or computed by PubChem | |
Canonical SMILES |
C(CCCl)CC(=O)Cl | |
Source | PubChem | |
URL | https://pubchem.ncbi.nlm.nih.gov | |
Description | Data deposited in or computed by PubChem | |
Molecular Formula |
C5H8Cl2O | |
Source | PubChem | |
URL | https://pubchem.ncbi.nlm.nih.gov | |
Description | Data deposited in or computed by PubChem | |
DSSTOX Substance ID |
DTXSID60166272 | |
Record name | Valeryl chloride, 5-chloro- | |
Source | EPA DSSTox | |
URL | https://comptox.epa.gov/dashboard/DTXSID60166272 | |
Description | DSSTox provides a high quality public chemistry resource for supporting improved predictive toxicology. | |
Molecular Weight |
155.02 g/mol | |
Source | PubChem | |
URL | https://pubchem.ncbi.nlm.nih.gov | |
Description | Data deposited in or computed by PubChem | |
CAS No. |
1575-61-7 | |
Record name | 5-Chlorovaleryl chloride | |
Source | CAS Common Chemistry | |
URL | https://commonchemistry.cas.org/detail?cas_rn=1575-61-7 | |
Description | CAS Common Chemistry is an open community resource for accessing chemical information. Nearly 500,000 chemical substances from CAS REGISTRY cover areas of community interest, including common and frequently regulated chemicals, and those relevant to high school and undergraduate chemistry classes. This chemical information, curated by our expert scientists, is provided in alignment with our mission as a division of the American Chemical Society. | |
Explanation | The data from CAS Common Chemistry is provided under a CC-BY-NC 4.0 license, unless otherwise stated. | |
Record name | Valeryl chloride, 5-chloro- | |
Source | ChemIDplus | |
URL | https://pubchem.ncbi.nlm.nih.gov/substance/?source=chemidplus&sourceid=0001575617 | |
Description | ChemIDplus is a free, web search system that provides access to the structure and nomenclature authority files used for the identification of chemical substances cited in National Library of Medicine (NLM) databases, including the TOXNET system. | |
Record name | 5-Chlorovaleryl chloride | |
Source | DTP/NCI | |
URL | https://dtp.cancer.gov/dtpstandard/servlet/dwindex?searchtype=NSC&outputformat=html&searchlist=84182 | |
Description | The NCI Development Therapeutics Program (DTP) provides services and resources to the academic and private-sector research communities worldwide to facilitate the discovery and development of new cancer therapeutic agents. | |
Explanation | Unless otherwise indicated, all text within NCI products is free of copyright and may be reused without our permission. Credit the National Cancer Institute as the source. | |
Record name | Valeryl chloride, 5-chloro- | |
Source | EPA DSSTox | |
URL | https://comptox.epa.gov/dashboard/DTXSID60166272 | |
Description | DSSTox provides a high quality public chemistry resource for supporting improved predictive toxicology. | |
Record name | 5-chlorovaleryl chloride | |
Source | European Chemicals Agency (ECHA) | |
URL | https://echa.europa.eu/substance-information/-/substanceinfo/100.014.913 | |
Description | The European Chemicals Agency (ECHA) is an agency of the European Union which is the driving force among regulatory authorities in implementing the EU's groundbreaking chemicals legislation for the benefit of human health and the environment as well as for innovation and competitiveness. | |
Explanation | Use of the information, documents and data from the ECHA website is subject to the terms and conditions of this Legal Notice, and subject to other binding limitations provided for under applicable law, the information, documents and data made available on the ECHA website may be reproduced, distributed and/or used, totally or in part, for non-commercial purposes provided that ECHA is acknowledged as the source: "Source: European Chemicals Agency, http://echa.europa.eu/". Such acknowledgement must be included in each copy of the material. ECHA permits and encourages organisations and individuals to create links to the ECHA website under the following cumulative conditions: Links can only be made to webpages that provide a link to the Legal Notice page. | |
Retrosynthesis Analysis
AI-Powered Synthesis Planning: Our tool employs the Template_relevance Pistachio, Template_relevance Bkms_metabolic, Template_relevance Pistachio_ringbreaker, Template_relevance Reaxys, Template_relevance Reaxys_biocatalysis model, leveraging a vast database of chemical reactions to predict feasible synthetic routes.
One-Step Synthesis Focus: Specifically designed for one-step synthesis, it provides concise and direct routes for your target compounds, streamlining the synthesis process.
Accurate Predictions: Utilizing the extensive PISTACHIO, BKMS_METABOLIC, PISTACHIO_RINGBREAKER, REAXYS, REAXYS_BIOCATALYSIS database, our tool offers high-accuracy predictions, reflecting the latest in chemical research and data.
Strategy Settings
Precursor scoring | Relevance Heuristic |
---|---|
Min. plausibility | 0.01 |
Model | Template_relevance |
Template Set | Pistachio/Bkms_metabolic/Pistachio_ringbreaker/Reaxys/Reaxys_biocatalysis |
Top-N result to add to graph | 6 |
Feasible Synthetic Routes
Q1: What are some of the synthetic applications of 5-Chlorovaleryl chloride?
A1: this compound serves as a key building block in various organic syntheses. For example, it acts as a starting material in the production of pyraclonil, a herbicide. The synthesis involves reacting this compound with malononitrile, formate, trichloroethylene, hydrazine hydrate, and p-toluenesulfonic acid propargyl alcohol ester through a seven-step process. Additionally, this compound plays a crucial role in synthesizing 1,1,7-Trichloro-1-hepten-3-one, a vital intermediate for pyrazole herbicides. This synthesis involves hydrolyzing and acylating 5-chloroamyl nitrile to obtain this compound, followed by addition and elimination reactions with 1,1-dichloroethylene.
Q2: Can this compound be used to synthesize organometallic compounds?
A2: Yes, this compound can be utilized in the synthesis of organometallic complexes containing cyclic carbenes. For instance, reacting this compound with [Mn(CO)5]– yields a low yield of the 2-oxacyclohexylidene complex cis-[Mn(I)[graphic omitted]H2(CO)4], demonstrating its potential in organometallic chemistry.
Q3: Are there any advantages to the synthetic routes utilizing this compound described in the research?
A3: The research highlights several advantages of using this compound in specific syntheses. For instance, the synthesis of pyraclonil using this compound offers a shorter route compared to existing methods, reducing the process by three reactions. This shortened route improves the overall yield and effectively lowers production costs, making it a more efficient approach. Similarly, the synthesis of 1,1,7-Trichloro-1-hepten-3-one using this compound results in high product purity (over 99%) and a good yield (85.9%).
Q4: How is 1,1,7-Trichloro-1-hepten-3-one, synthesized from this compound, characterized?
A4: Following the synthesis of 1,1,7-Trichloro-1-hepten-3-one from this compound, the final product is characterized using spectroscopic techniques. Specifically, infrared spectroscopy (IR) and proton nuclear magnetic resonance spectroscopy (1H NMR) are employed to confirm the product's identity and analyze its structural features.
Disclaimer and Information on In-Vitro Research Products
Please be aware that all articles and product information presented on BenchChem are intended solely for informational purposes. The products available for purchase on BenchChem are specifically designed for in-vitro studies, which are conducted outside of living organisms. In-vitro studies, derived from the Latin term "in glass," involve experiments performed in controlled laboratory settings using cells or tissues. It is important to note that these products are not categorized as medicines or drugs, and they have not received approval from the FDA for the prevention, treatment, or cure of any medical condition, ailment, or disease. We must emphasize that any form of bodily introduction of these products into humans or animals is strictly prohibited by law. It is essential to adhere to these guidelines to ensure compliance with legal and ethical standards in research and experimentation.