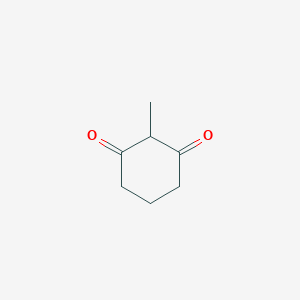
2-Methylcyclohexane-1,3-dione
Overview
Description
2-Methylcyclohexane-1,3-dione is a synthetic intermediate . It is an important starting material, exemplified in a formal synthesis of acorone . It is also used in Organic Synthesis, Pharmaceuticals, Agrochemicals .
Synthesis Analysis
Cyclohexane-1,3-dione derivatives are considered as a key structural precursor for the synthesis of synthetically significant compounds such as 4H-chromenones, 2H-xanthenones, coumarins, enaminones, acridinedione, 1,4-dihydropyridine, different other heterocycles, and natural products .Molecular Structure Analysis
The molecular formula of 2-Methylcyclohexane-1,3-dione is C7H10O2 . It has 19 bonds - 9 non-H bonds, 2 multiple bonds, 2 double bonds, 1 six-membered ring, and 2 ketones (aliphatic) .Chemical Reactions Analysis
2-Methylcyclohexane-1,3-dione acts as a synthetic intermediate . It is an important starting material, exemplified in a formal synthesis of acorone . It is also used in Organic Synthesis, Pharmaceuticals, Agrochemicals .Physical And Chemical Properties Analysis
The physical and chemical properties of 2-Methylcyclohexane-1,3-dione include a density of 1.1±0.1 g/cm3, boiling point of 235.7±23.0 °C at 760 mmHg, vapour pressure of 0.0±0.5 mmHg at 25°C, enthalpy of vaporization of 47.2±3.0 kJ/mol, flash point of 85.9±19.6 °C, index of refraction of 1.459, molar refractivity of 32.6±0.3 cm3 .Scientific Research Applications
Synthetic Intermediate
2-Methylcyclohexane-1,3-dione acts as a synthetic intermediate . This means it is used in the production of other compounds in a multi-step synthesis process. It plays a crucial role in the formation of complex molecules from simpler ones.
Starting Material
It is an important starting material, exemplified in a formal synthesis of acorone . Acorone is a naturally occurring compound found in certain plants and has been studied for its potential medicinal properties.
Organic Synthesis
2-Methylcyclohexane-1,3-dione is used in organic synthesis . Organic synthesis is a method of preparation of organic compounds. It is a critical process in the development of pharmaceuticals, agrochemicals, and many other types of organic products.
Pharmaceuticals
This compound is used in the pharmaceutical industry . It could be a precursor to active pharmaceutical ingredients or used in the synthesis of pharmaceutical intermediates.
Agrochemicals
2-Methylcyclohexane-1,3-dione is also used in the production of agrochemicals . These are chemicals such as pesticides, herbicides, and fertilizers that help in crop protection and enhancement.
Solubility
It is soluble in methanol . This property can be exploited in various chemical reactions and processes where a methanol solvent is used.
Safety and Hazards
2-Methylcyclohexane-1,3-dione may cause skin irritation, serious eye irritation, and respiratory irritation . It is advised to wear personal protective equipment/face protection, ensure adequate ventilation, avoid getting in eyes, on skin, or on clothing, avoid ingestion and inhalation, and avoid dust formation .
Mechanism of Action
Target of Action
It is known to act as a synthetic intermediate in various chemical reactions .
Mode of Action
2-Methylcyclohexane-1,3-dione interacts with its targets through chemical reactions, serving as an important starting material. It is used in the formal synthesis of acorone . The exact mode of interaction with its targets is subject to the specific reaction conditions and the nature of the other reactants involved.
Biochemical Pathways
It is known to be involved in the synthesis of various downstream products, including derivatives of 2,6-dihydroxytoluene, which are widely used in the preparation of cosmetics .
Result of Action
The molecular and cellular effects of 2-Methylcyclohexane-1,3-dione’s action would depend on the specific context of its use. As a synthetic intermediate, its primary role is to participate in chemical reactions to produce desired end products .
Action Environment
Environmental factors can influence the action, efficacy, and stability of 2-Methylcyclohexane-1,3-dione. For instance, it should be stored in an inert atmosphere at room temperature . Its reactivity may also be influenced by factors such as pH, temperature, and the presence of other substances in the reaction environment.
properties
IUPAC Name |
2-methylcyclohexane-1,3-dione | |
---|---|---|
Source | PubChem | |
URL | https://pubchem.ncbi.nlm.nih.gov | |
Description | Data deposited in or computed by PubChem | |
InChI |
InChI=1S/C7H10O2/c1-5-6(8)3-2-4-7(5)9/h5H,2-4H2,1H3 | |
Source | PubChem | |
URL | https://pubchem.ncbi.nlm.nih.gov | |
Description | Data deposited in or computed by PubChem | |
InChI Key |
VSGJHHIAMHUZKF-UHFFFAOYSA-N | |
Source | PubChem | |
URL | https://pubchem.ncbi.nlm.nih.gov | |
Description | Data deposited in or computed by PubChem | |
Canonical SMILES |
CC1C(=O)CCCC1=O | |
Source | PubChem | |
URL | https://pubchem.ncbi.nlm.nih.gov | |
Description | Data deposited in or computed by PubChem | |
Molecular Formula |
C7H10O2 | |
Source | PubChem | |
URL | https://pubchem.ncbi.nlm.nih.gov | |
Description | Data deposited in or computed by PubChem | |
DSSTOX Substance ID |
DTXSID90152406 | |
Record name | 2-Methylcyclohexane-1,3-dione | |
Source | EPA DSSTox | |
URL | https://comptox.epa.gov/dashboard/DTXSID90152406 | |
Description | DSSTox provides a high quality public chemistry resource for supporting improved predictive toxicology. | |
Molecular Weight |
126.15 g/mol | |
Source | PubChem | |
URL | https://pubchem.ncbi.nlm.nih.gov | |
Description | Data deposited in or computed by PubChem | |
Product Name |
2-Methylcyclohexane-1,3-dione | |
CAS RN |
1193-55-1 | |
Record name | 2-Methyl-1,3-cyclohexanedione | |
Source | CAS Common Chemistry | |
URL | https://commonchemistry.cas.org/detail?cas_rn=1193-55-1 | |
Description | CAS Common Chemistry is an open community resource for accessing chemical information. Nearly 500,000 chemical substances from CAS REGISTRY cover areas of community interest, including common and frequently regulated chemicals, and those relevant to high school and undergraduate chemistry classes. This chemical information, curated by our expert scientists, is provided in alignment with our mission as a division of the American Chemical Society. | |
Explanation | The data from CAS Common Chemistry is provided under a CC-BY-NC 4.0 license, unless otherwise stated. | |
Record name | 2-Methylcyclohexane-1,3-dione | |
Source | ChemIDplus | |
URL | https://pubchem.ncbi.nlm.nih.gov/substance/?source=chemidplus&sourceid=0001193551 | |
Description | ChemIDplus is a free, web search system that provides access to the structure and nomenclature authority files used for the identification of chemical substances cited in National Library of Medicine (NLM) databases, including the TOXNET system. | |
Record name | 1193-55-1 | |
Source | DTP/NCI | |
URL | https://dtp.cancer.gov/dtpstandard/servlet/dwindex?searchtype=NSC&outputformat=html&searchlist=54460 | |
Description | The NCI Development Therapeutics Program (DTP) provides services and resources to the academic and private-sector research communities worldwide to facilitate the discovery and development of new cancer therapeutic agents. | |
Explanation | Unless otherwise indicated, all text within NCI products is free of copyright and may be reused without our permission. Credit the National Cancer Institute as the source. | |
Record name | 2-Methylcyclohexane-1,3-dione | |
Source | EPA DSSTox | |
URL | https://comptox.epa.gov/dashboard/DTXSID90152406 | |
Description | DSSTox provides a high quality public chemistry resource for supporting improved predictive toxicology. | |
Record name | 2-methylcyclohexane-1,3-dione | |
Source | European Chemicals Agency (ECHA) | |
URL | https://echa.europa.eu/substance-information/-/substanceinfo/100.013.431 | |
Description | The European Chemicals Agency (ECHA) is an agency of the European Union which is the driving force among regulatory authorities in implementing the EU's groundbreaking chemicals legislation for the benefit of human health and the environment as well as for innovation and competitiveness. | |
Explanation | Use of the information, documents and data from the ECHA website is subject to the terms and conditions of this Legal Notice, and subject to other binding limitations provided for under applicable law, the information, documents and data made available on the ECHA website may be reproduced, distributed and/or used, totally or in part, for non-commercial purposes provided that ECHA is acknowledged as the source: "Source: European Chemicals Agency, http://echa.europa.eu/". Such acknowledgement must be included in each copy of the material. ECHA permits and encourages organisations and individuals to create links to the ECHA website under the following cumulative conditions: Links can only be made to webpages that provide a link to the Legal Notice page. | |
Synthesis routes and methods
Procedure details
Retrosynthesis Analysis
AI-Powered Synthesis Planning: Our tool employs the Template_relevance Pistachio, Template_relevance Bkms_metabolic, Template_relevance Pistachio_ringbreaker, Template_relevance Reaxys, Template_relevance Reaxys_biocatalysis model, leveraging a vast database of chemical reactions to predict feasible synthetic routes.
One-Step Synthesis Focus: Specifically designed for one-step synthesis, it provides concise and direct routes for your target compounds, streamlining the synthesis process.
Accurate Predictions: Utilizing the extensive PISTACHIO, BKMS_METABOLIC, PISTACHIO_RINGBREAKER, REAXYS, REAXYS_BIOCATALYSIS database, our tool offers high-accuracy predictions, reflecting the latest in chemical research and data.
Strategy Settings
Precursor scoring | Relevance Heuristic |
---|---|
Min. plausibility | 0.01 |
Model | Template_relevance |
Template Set | Pistachio/Bkms_metabolic/Pistachio_ringbreaker/Reaxys/Reaxys_biocatalysis |
Top-N result to add to graph | 6 |
Feasible Synthetic Routes
Q & A
Q1: What are the common synthetic routes to 2-Methylcyclohexane-1,3-dione?
A1: One common approach starts with resorcinol, which undergoes high-pressure reduction followed by methylation. [] Alternatively, 2-Methylcyclohexane-1,3-dione can be synthesized through the alkylation of the potassium salt of cyclohexane-1,3-dione with prop-2-ynyl bromide in dimethylformamide. []
Q2: How does the structure of 2-Methylcyclohexane-1,3-dione influence its reactivity?
A2: The presence of two carbonyl groups makes 2-Methylcyclohexane-1,3-dione a β-diketone. This structural feature allows it to participate in various reactions, including alkylation, Michael additions, and condensations. [, , , , , ] For instance, it readily undergoes C-selective alkylation with unactivated sp3 electrophiles when protected as a ketodimethyl hydrazone. []
Q3: How does 2-Methylcyclohexane-1,3-dione behave in Michael additions?
A4: 2-Methylcyclohexane-1,3-dione acts as a Michael donor in reactions with electron-deficient alkenes. For example, it reacts with β-vinylbutenolide via a 1,6-Michael addition followed by cyclization. [] Another example is its reaction with methyl vinyl ketone, a crucial step in synthesizing 9-Methyl-5(10)-octalin-1,6-dione, a valuable intermediate in terpenoid and steroid synthesis. []
Q4: Are there any catalytic applications of 2-Methylcyclohexane-1,3-dione?
A5: While 2-Methylcyclohexane-1,3-dione is primarily used as a building block, its reaction with methyl vinyl ketone can be catalyzed by potassium-modified ZrO2. This reaction highlights the potential for developing heterogeneous catalytic systems for synthesizing complex molecules from 2-Methylcyclohexane-1,3-dione. [, ]
Q5: What are the challenges associated with using 2-Methylcyclohexane-1,3-dione in liquid-phase reactions?
A6: One study observed that potassium-modified ZrO2 catalysts, while effective in the vapor phase, can leach potassium in liquid-phase reactions, limiting their practicality for such applications. []
Q6: Can you provide examples of natural products or bioactive compounds synthesized using 2-Methylcyclohexane-1,3-dione as a starting material?
A7: 2-Methylcyclohexane-1,3-dione is a key intermediate in synthesizing various natural products. One example is its use in the synthesis of Stachybotrin D, a potential anti-HIV drug derived from a sponge-derived fungus. [] Another study utilized 2-Methylcyclohexane-1,3-dione in synthesizing (S)‐8a‐Methyl‐3,4,8,8a‐Tetrahydro‐1,6‐(2H,7H)‐Naphthalenedione, a Wieland Miescher ketone (WMK) precursor, further highlighting its versatility in natural product synthesis. []
Q7: Have there been any investigations into the base-induced reactions of 2-Methylcyclohexane-1,3-dione?
A8: Yes, researchers have studied the reactions of 2-Methylcyclohexane-1,3-dione derivatives, such as 2-bromo- and 2-chloro-2-methylcyclohexane-1,3-dione, with bases like sodium acetate in various solvents. These studies revealed that the reaction outcome is highly dependent on the specific 2-halogeno-1,3-diketone used and the solvent system, providing insights into the reaction mechanisms and potential synthetic applications. []
Disclaimer and Information on In-Vitro Research Products
Please be aware that all articles and product information presented on BenchChem are intended solely for informational purposes. The products available for purchase on BenchChem are specifically designed for in-vitro studies, which are conducted outside of living organisms. In-vitro studies, derived from the Latin term "in glass," involve experiments performed in controlled laboratory settings using cells or tissues. It is important to note that these products are not categorized as medicines or drugs, and they have not received approval from the FDA for the prevention, treatment, or cure of any medical condition, ailment, or disease. We must emphasize that any form of bodily introduction of these products into humans or animals is strictly prohibited by law. It is essential to adhere to these guidelines to ensure compliance with legal and ethical standards in research and experimentation.