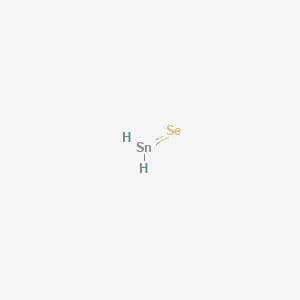
Tin selenide (SnSe)
Overview
Description
Tin selenide (SnSe) is a narrow band gap semiconductor comprised of environmentally friendly and earth abundant elements . It has recently proven to be an extraordinarily promising thermoelectric material with intrinsically ultralow lattice thermal conductivity . It is also used in a variety of applications, such as ion-selective sensors, solar absorption, solar control coatings, and diodes .
Synthesis Analysis
SnSe anodes are synthesized by a mechanochemical route employing a high-energy ball mill without the addition of carbon during the synthesis . A simple approach to synthesizing tin selenide nanostructures showing superior photocatalytic performance with variable tin ratios via a facile chemical method is also reported .Molecular Structure Analysis
SnSe is made up of tightly bound layers formed by double planes each of which consists of zigzag chains of tin and selenium atoms . At room temperature, SnSe adopts an orthorhombic crystal structure .Chemical Reactions Analysis
High-temperature oxidation of SnSe in the temperature interval 25 to 650‡ C has been studied by X-ray photoelectron spectroscopy, X-ray diffraction, and thermal analysis techniques . Exposure to dry oxygen (760 torr pressure) at up to 200‡ C leads to the formation of SnO 2 on the surface .Physical And Chemical Properties Analysis
Tin selenide (SnSe) is a member of (IVA–VIA) binary semiconductors family and has a high absorption coefficient . The bandgap of SnSe varies from 0.9 eV to 1.3 eV . SnSe has an orthorhombic crystal structure, and it has p-type conductivity with Sn-vacancies .Scientific Research Applications
Solar Cells
Tin Selenide is used in the production of solar cells due to its optimal band gap . The material’s properties make it an excellent choice for converting sunlight into electricity, contributing to the development of renewable energy sources .
Photodetectors
SnSe is also used in photodetectors . These devices convert light into an electrical current. The optimal band gap of SnSe makes it suitable for detecting a wide range of light wavelengths .
Memory Devices
Tin Selenide is used in memory devices . Its unique properties allow it to store data efficiently, making it a valuable material in the field of data storage and retrieval .
Lithium and Sodium-ion Batteries
SnSe is used as an anode material for lithium and sodium-ion batteries . Its properties contribute to the batteries’ high capacity and long cycle life .
Gas Sensing
Tin Selenide is used in gas sensing applications . It can detect various gases, making it useful in environmental monitoring and safety applications .
Photocatalysis
SnSe is used in photocatalysis . It can absorb light and use the energy to drive chemical reactions, making it useful in various industrial processes .
Supercapacitors
Tin Selenide is used in supercapacitors . These devices store and release energy quickly, making them useful in applications that require rapid energy discharge .
Topological Insulators and Resistive Switching Devices
Lastly, SnSe is used in topological insulators and resistive switching devices due to its optimal band gap . These applications are crucial in the field of electronics and information technology .
Each of these applications leverages the unique properties of Tin Selenide, making it a versatile and valuable material in scientific research and various industries .
Mechanism of Action
Target of Action
Tin Selenide primarily targets various fields of applied material science . It has demonstrated potential in applications such as solar cells , photodetectors , memory devices , lithium and sodium-ion batteries , gas sensing , photocatalysis , supercapacitors , topological insulators , and resistive switching devices . These applications strongly depend on the properties of SnSe, including optical, electrical, and microstructural properties .
Mode of Action
The mode of action of Tin Selenide is primarily through its optimal band gap . In thermoelectric applications, Tin Selenide can directly convert waste heat to electrical energy . In photodetectors, it exhibits a fast response speed with good responsivity and detectivity in the NIR range .
Biochemical Pathways
For instance, its use in solar cells and batteries contributes to energy production and storage . Its role in gas sensing and photocatalysis can influence environmental monitoring and pollution control .
Pharmacokinetics
The synthesis and deposition methods of tin selenide play an essential role in obtaining high-quality materials .
Result of Action
The result of Tin Selenide’s action is evident in its various applications. For example, in thermoelectric materials, it can pave the way to reuse waste energy . In photodetectors, it has exhibited fast response speed with good responsivity and detectivity in the NIR range .
Action Environment
The action of Tin Selenide can be influenced by environmental factors. For instance, the synthesis and deposition methods, which are crucial for obtaining high-quality materials, can be affected by the working environment . Furthermore, the performance of Tin Selenide in applications like solar cells and photodetectors can be influenced by factors such as light intensity and temperature .
Safety and Hazards
Future Directions
Tin selenide has demonstrated great potential in the applied material science . It exists in two phases, i.e., SnSe and SnSe2, and has demonstrated versatility in thermoelectric, photodetector, solar cells, photocatalytic, phase change memory, gas sensing, anode material for battery, supercapacitor, and topological insulator . These applications strongly depend upon the properties of SnSe (optical, electrical, and microstructural, etc.) . Apart from material properties, material synthesis/deposition methods also play an essential role in obtaining high-quality materials .
properties
IUPAC Name |
selanylidenestannane | |
---|---|---|
Source | PubChem | |
URL | https://pubchem.ncbi.nlm.nih.gov | |
Description | Data deposited in or computed by PubChem | |
InChI |
InChI=1S/Se.Sn.2H | |
Source | PubChem | |
URL | https://pubchem.ncbi.nlm.nih.gov | |
Description | Data deposited in or computed by PubChem | |
InChI Key |
RTZUOCZUTSLBGC-UHFFFAOYSA-N | |
Source | PubChem | |
URL | https://pubchem.ncbi.nlm.nih.gov | |
Description | Data deposited in or computed by PubChem | |
Canonical SMILES |
[Se]=[SnH2] | |
Source | PubChem | |
URL | https://pubchem.ncbi.nlm.nih.gov | |
Description | Data deposited in or computed by PubChem | |
Molecular Formula |
H2SeSn | |
Source | PubChem | |
URL | https://pubchem.ncbi.nlm.nih.gov | |
Description | Data deposited in or computed by PubChem | |
Molecular Weight |
199.70 g/mol | |
Source | PubChem | |
URL | https://pubchem.ncbi.nlm.nih.gov | |
Description | Data deposited in or computed by PubChem | |
Product Name |
Tin selenide (SnSe) | |
CAS RN |
1315-06-6 | |
Record name | Stannous selenide | |
Source | ChemIDplus | |
URL | https://pubchem.ncbi.nlm.nih.gov/substance/?source=chemidplus&sourceid=0001315066 | |
Description | ChemIDplus is a free, web search system that provides access to the structure and nomenclature authority files used for the identification of chemical substances cited in National Library of Medicine (NLM) databases, including the TOXNET system. | |
Q & A
Q1: What is the molecular formula and weight of tin selenide?
A1: The molecular formula of tin selenide is SnSe. Its molecular weight is 197.57 g/mol.
Q2: What is the crystal structure of SnSe?
A2: Tin selenide commonly exists in an orthorhombic crystal structure (Pnma) at ambient conditions. [] This structure contributes to its anisotropic properties, meaning its properties differ along different crystallographic axes. It can also exist in a cubic rock-salt structure (Fm3̄m), but this phase is typically stabilized under high pressure. []
Q3: What is the significance of the anisotropic nature of SnSe?
A3: The anisotropic nature of SnSe significantly impacts its thermal and electrical properties. For instance, its thermal conductivity is significantly lower in the cross-plane direction compared to the in-plane direction. [] This anisotropy makes SnSe particularly interesting for thermoelectric applications, where directional control of heat and charge flow is crucial. []
Q4: What is the bandgap of SnSe, and how does it impact its applications?
A4: SnSe is classified as a semiconductor. [] The bandgap of SnSe has been reported to range from 0.79 eV to 3.2 eV depending on the preparation method and the structure of the material. [, ] This range allows for potential applications in various fields, including photovoltaics (solar cells), where efficient absorption of sunlight is crucial, and thermoelectrics, where a balance between electrical conductivity and Seebeck coefficient is desired. [, ]
Q5: How does the optical absorption of SnSe vary with its crystal orientation?
A5: SnSe exhibits anisotropic optical absorption due to its puckered in-plane lattice structure. [] This means that the material's absorption of light changes depending on the angle of incident light with respect to its crystal axes. This property has implications for the development of polarized light detectors and other optoelectronic devices. [, ]
Q6: What methods are commonly employed for synthesizing SnSe?
A6: SnSe can be synthesized using various techniques, including:
- Direct Vapor Transport (DVT): This method involves vaporizing SnSe powder and then condensing it onto a substrate, resulting in single crystals. []
- Thermal Evaporation: This method involves heating SnSe powder under vacuum, causing it to evaporate and deposit onto a substrate, forming thin films. [, , ]
- Chemical Bath Deposition: This is a solution-based method where SnSe is deposited onto a substrate from a chemical bath containing precursor materials. []
- Mechanical Alloying: This method involves grinding elemental powders of tin and selenium together to form SnSe powder. []
- Solution-Phase Synthesis: This approach involves reactions in a solvent to produce SnSe nanostructures with controlled size and morphology. []
Q7: How does the synthesis method affect the properties of SnSe?
A7: The synthesis method significantly influences the properties of SnSe. For example, thin films prepared by thermal evaporation often have different properties compared to bulk single crystals grown by DVT. [] The choice of method depends on the desired application and the specific properties required. []
Q8: What characterization techniques are used to analyze SnSe materials?
A8: Several techniques are used to characterize SnSe materials, including:
- X-ray diffraction (XRD): This technique is used to determine the crystal structure, phase purity, and crystallite size of SnSe. [, , ]
- Scanning electron microscopy (SEM): This technique is used to image the surface morphology and analyze the size and shape of SnSe particles or films. [, ]
- Atomic force microscopy (AFM): This technique provides high-resolution images of the surface topography and can be used to study the nanoscale morphology and mechanical properties of SnSe. [, ]
- UV-Vis Spectroscopy: This technique is used to determine the optical properties of SnSe, particularly its band gap, by analyzing the absorption and transmission of light through the material. [, , , , ]
- Raman Spectroscopy: This technique provides information about the vibrational modes of molecules in SnSe, which can be used to identify the material and study its crystal structure and defects. [, ]
- Four-Point Probe & Hall Effect Measurements: These techniques are used to determine the electrical properties of SnSe, such as its electrical conductivity, carrier concentration, and mobility. [, , , ]
Q9: What makes SnSe a promising material for thermoelectric applications?
A9: SnSe possesses several properties that make it attractive for thermoelectric applications:
- Ultralow thermal conductivity: SnSe exhibits remarkably low thermal conductivity, especially in its single crystal form, due to strong anharmonicity in its lattice vibrations. [, , , , ] This property is crucial for achieving a high thermoelectric figure of merit (ZT).
- Moderate electrical conductivity: SnSe exhibits moderate electrical conductivity, which can be further enhanced by doping. [, ] This balance between electrical and thermal properties is essential for efficient thermoelectric energy conversion.
- Earth-abundant and environmentally friendly: Unlike some other high-performance thermoelectric materials, SnSe is composed of elements that are abundant and non-toxic, making it a sustainable choice. [, ]
Q10: How does doping affect the thermoelectric performance of SnSe?
A10: Doping can significantly enhance the thermoelectric performance of SnSe by optimizing its electrical properties:
- Increased carrier concentration: Introducing dopants can increase the concentration of charge carriers (electrons or holes), leading to higher electrical conductivity. [, , ]
- Tuning the Fermi level: Doping can shift the Fermi level, influencing the Seebeck coefficient and potentially enhancing the power factor. [, ]
- Scattering phonons: Dopant atoms can scatter phonons, further reducing thermal conductivity and contributing to a higher ZT. [, ]
Q11: What are the advantages of using nanostructured SnSe for thermoelectrics?
A11: Nanostructuring SnSe, such as creating nanosheets or nanocrystals, can further enhance its thermoelectric properties:
- Reduced thermal conductivity: Increased phonon scattering at grain boundaries and interfaces in nanostructured SnSe leads to further reductions in thermal conductivity. [, , ]
- Enhanced power factor: Nanostructuring can influence the electronic band structure and carrier scattering, potentially leading to an increased power factor. []
Q12: What other applications, besides thermoelectrics, is SnSe being explored for?
A12: In addition to its promising thermoelectric properties, SnSe is being investigated for various other applications, including:
- Photovoltaics: SnSe's suitable band gap and good absorption coefficient make it a potential candidate for solar cell applications. [, , , ]
- Lithium-ion batteries: SnSe has shown potential as an anode material for lithium-ion batteries due to its good electrochemical performance. []
- Photodetectors: The anisotropic optical properties and high sensitivity of SnSe make it promising for photodetector applications, particularly in the UV-visible-NIR range. [, ]
Q13: What are the limitations or challenges associated with SnSe research and applications?
A13: Despite its promising properties, there are still challenges to overcome in SnSe research and development:
- Scalability and cost: Synthesizing high-quality single crystals of SnSe can be expensive and challenging to scale up for large-scale applications. []
- Mechanical stability: SnSe single crystals can be relatively brittle, which may pose challenges for device fabrication and long-term reliability. []
- Air stability: SnSe can be susceptible to oxidation in air, which could degrade its performance over time. []
Q14: How is computational chemistry being utilized in SnSe research?
A14: Computational chemistry plays a crucial role in understanding and predicting the properties of SnSe:
- First-principles calculations: Density functional theory (DFT) calculations are employed to study the electronic band structure, phonon dispersion, and thermal conductivity of SnSe. [, , , ]
- Modeling doping effects: Computational methods help to predict the impact of different dopants on the thermoelectric properties of SnSe. [, ]
- Simulating nanostructures: Computational models can simulate the properties of SnSe nanostructures, providing insights into their enhanced performance. []
Q15: What are the future directions and research opportunities in the field of SnSe?
A15: The field of SnSe research continues to evolve, with several promising avenues for future exploration:
- Optimizing doping strategies: Developing more effective doping techniques to further enhance the thermoelectric performance of SnSe remains an active area of research. []
- Exploring new synthesis methods: Finding scalable and cost-effective methods for producing high-quality SnSe materials is crucial for commercialization. []
- Developing novel device architectures: Designing innovative device structures that exploit the anisotropic properties of SnSe for enhanced performance is an exciting direction for future research. []
- Expanding applications: Investigating the potential of SnSe in emerging fields such as flexible electronics, energy storage beyond lithium-ion batteries, and sensing applications will further broaden its impact. [, ]
Disclaimer and Information on In-Vitro Research Products
Please be aware that all articles and product information presented on BenchChem are intended solely for informational purposes. The products available for purchase on BenchChem are specifically designed for in-vitro studies, which are conducted outside of living organisms. In-vitro studies, derived from the Latin term "in glass," involve experiments performed in controlled laboratory settings using cells or tissues. It is important to note that these products are not categorized as medicines or drugs, and they have not received approval from the FDA for the prevention, treatment, or cure of any medical condition, ailment, or disease. We must emphasize that any form of bodily introduction of these products into humans or animals is strictly prohibited by law. It is essential to adhere to these guidelines to ensure compliance with legal and ethical standards in research and experimentation.