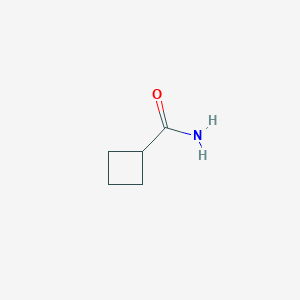
Cyclobutanecarboxamide
Overview
Description
Cyclobutanecarboxamide is an organic compound with the molecular formula C₅H₉NO. It is a derivative of cyclobutane, featuring a carboxamide functional group. This compound is known for its stability and solubility in water and most organic solvents. This compound appears as a colorless to pale yellow liquid and is used in various chemical synthesis processes .
Mechanism of Action
Target of Action
Cyclobutanecarboxamide is an organic compound
Biochemical Pathways
This compound may participate in various biochemical reactions as an intermediate . It can be involved in the synthesis of other organic compounds such as amides, amines, aldehydes, and ketones . .
Action Environment
The action, efficacy, and stability of this compound can be influenced by various environmental factors. For instance, the compound is stable at room temperature . It’s soluble in water and most organic solvents , which could influence its distribution in different environments. Safety measures should be taken while handling this compound as it may cause irritation to eyes, skin, and respiratory tract .
Preparation Methods
Cyclobutanecarboxamide can be synthesized through several methods. One common synthetic route involves the reaction of cyclobutanecarboxylic acid with ammonia. The process typically involves the following steps :
Cyclobutanecarboxylic acid: is dissolved in dry tetrahydrofuran.
N-methylmorpholine: is added to the solution, followed by cooling to -15°C.
Ethyl chloroformate: is introduced, causing the formation of a white precipitate.
Anhydrous ammonia: is bubbled through the solution, resulting in the formation of this compound.
This method yields this compound with a high degree of purity and efficiency. Industrial production methods may involve similar steps but on a larger scale, with optimized reaction conditions to maximize yield and minimize costs .
Chemical Reactions Analysis
Cyclobutanecarboxamide undergoes various chemical reactions, including:
Oxidation: this compound can be oxidized to form cyclobutanecarboxylic acid using oxidizing agents such as potassium permanganate.
Reduction: It can be reduced to cyclobutylamine using reducing agents like lithium aluminum hydride.
Substitution: this compound can participate in substitution reactions, where the carboxamide group is replaced by other functional groups under specific conditions.
Common reagents used in these reactions include potassium permanganate for oxidation, lithium aluminum hydride for reduction, and various halogenating agents for substitution reactions. The major products formed from these reactions include cyclobutanecarboxylic acid, cyclobutylamine, and substituted cyclobutane derivatives .
Scientific Research Applications
Cyclobutanecarboxamide has several scientific research applications:
Chemistry: It is used as an intermediate in organic synthesis, particularly in the preparation of other cyclobutane derivatives.
Biology: this compound derivatives are studied for their potential biological activities, including antimicrobial and anticancer properties.
Medicine: Research is ongoing to explore the therapeutic potential of this compound derivatives in treating various diseases.
Industry: It is used in the production of dyes, surfactants, and optical coatings
Comparison with Similar Compounds
Cyclobutanecarboxamide can be compared with other similar compounds, such as:
Cyclobutanecarboxylic acid: This compound is the oxidized form of this compound and shares similar structural features.
Cyclobutylamine: The reduced form of this compound, used in various chemical synthesis processes.
Cyclobutanone: Another cyclobutane derivative, used as an intermediate in organic synthesis.
This compound is unique due to its stability and versatility in chemical reactions, making it a valuable compound in both research and industrial applications .
Properties
IUPAC Name |
cyclobutanecarboxamide | |
---|---|---|
Source | PubChem | |
URL | https://pubchem.ncbi.nlm.nih.gov | |
Description | Data deposited in or computed by PubChem | |
InChI |
InChI=1S/C5H9NO/c6-5(7)4-2-1-3-4/h4H,1-3H2,(H2,6,7) | |
Source | PubChem | |
URL | https://pubchem.ncbi.nlm.nih.gov | |
Description | Data deposited in or computed by PubChem | |
InChI Key |
MFNYBOWJWGPXFM-UHFFFAOYSA-N | |
Source | PubChem | |
URL | https://pubchem.ncbi.nlm.nih.gov | |
Description | Data deposited in or computed by PubChem | |
Canonical SMILES |
C1CC(C1)C(=O)N | |
Source | PubChem | |
URL | https://pubchem.ncbi.nlm.nih.gov | |
Description | Data deposited in or computed by PubChem | |
Molecular Formula |
C5H9NO | |
Source | PubChem | |
URL | https://pubchem.ncbi.nlm.nih.gov | |
Description | Data deposited in or computed by PubChem | |
DSSTOX Substance ID |
DTXSID60361563 | |
Record name | cyclobutanecarboxamide | |
Source | EPA DSSTox | |
URL | https://comptox.epa.gov/dashboard/DTXSID60361563 | |
Description | DSSTox provides a high quality public chemistry resource for supporting improved predictive toxicology. | |
Molecular Weight |
99.13 g/mol | |
Source | PubChem | |
URL | https://pubchem.ncbi.nlm.nih.gov | |
Description | Data deposited in or computed by PubChem | |
CAS No. |
1503-98-6 | |
Record name | cyclobutanecarboxamide | |
Source | EPA DSSTox | |
URL | https://comptox.epa.gov/dashboard/DTXSID60361563 | |
Description | DSSTox provides a high quality public chemistry resource for supporting improved predictive toxicology. | |
Record name | Cyclobutanecarboxamide | |
Source | European Chemicals Agency (ECHA) | |
URL | https://echa.europa.eu/information-on-chemicals | |
Description | The European Chemicals Agency (ECHA) is an agency of the European Union which is the driving force among regulatory authorities in implementing the EU's groundbreaking chemicals legislation for the benefit of human health and the environment as well as for innovation and competitiveness. | |
Explanation | Use of the information, documents and data from the ECHA website is subject to the terms and conditions of this Legal Notice, and subject to other binding limitations provided for under applicable law, the information, documents and data made available on the ECHA website may be reproduced, distributed and/or used, totally or in part, for non-commercial purposes provided that ECHA is acknowledged as the source: "Source: European Chemicals Agency, http://echa.europa.eu/". Such acknowledgement must be included in each copy of the material. ECHA permits and encourages organisations and individuals to create links to the ECHA website under the following cumulative conditions: Links can only be made to webpages that provide a link to the Legal Notice page. | |
Synthesis routes and methods
Procedure details
Retrosynthesis Analysis
AI-Powered Synthesis Planning: Our tool employs the Template_relevance Pistachio, Template_relevance Bkms_metabolic, Template_relevance Pistachio_ringbreaker, Template_relevance Reaxys, Template_relevance Reaxys_biocatalysis model, leveraging a vast database of chemical reactions to predict feasible synthetic routes.
One-Step Synthesis Focus: Specifically designed for one-step synthesis, it provides concise and direct routes for your target compounds, streamlining the synthesis process.
Accurate Predictions: Utilizing the extensive PISTACHIO, BKMS_METABOLIC, PISTACHIO_RINGBREAKER, REAXYS, REAXYS_BIOCATALYSIS database, our tool offers high-accuracy predictions, reflecting the latest in chemical research and data.
Strategy Settings
Precursor scoring | Relevance Heuristic |
---|---|
Min. plausibility | 0.01 |
Model | Template_relevance |
Template Set | Pistachio/Bkms_metabolic/Pistachio_ringbreaker/Reaxys/Reaxys_biocatalysis |
Top-N result to add to graph | 6 |
Feasible Synthetic Routes
Q1: What is the primary use of Cyclobutanecarboxamide in current research?
A1: this compound serves as a versatile building block in organic synthesis and medicinal chemistry. Researchers use it as a starting material to create a diverse array of compounds, particularly those with potential therapeutic applications. [, , ]
Q2: How do structural modifications to this compound affect its interaction with melatonin receptors (MT1 and MT2)?
A2: Research indicates that even subtle changes to the this compound scaffold can profoundly impact its affinity and selectivity for melatonin receptors. For instance, introducing a 2-[(2,3-dihydro-1H-indol-1-yl)methyl] group led to compounds like 6b, exhibiting high affinity and selectivity for the MT2 subtype. Conversely, modifications like 5-Me, 5-OMe, 5-Br, 6-NH2, and 6-NO2 substitutions on the indoline moiety reduced both affinity and selectivity for MT2, highlighting the importance of hydrophobic interactions in this region. []
Q3: Can you elaborate on the role of this compound derivatives as histamine H3 receptor antagonists?
A3: Compounds like trans-N-ethyl-3-fluoro-3-[3-fluoro-4-(pyrrolidinylmethyl)phenyl]this compound (PF-03654746) and trans-3-fluoro-3-[3-fluoro-4-(pyrrolidin-1-ylmethyl)phenyl]-N-(2-methylpropyl)this compound (PF-03654764) exemplify this compound derivatives developed as potent and selective histamine H3 receptor antagonists. Extensive medicinal chemistry efforts, coupled with computational modeling and safety profiling, were crucial in identifying these clinical candidates with promising pharmacological and pharmacokinetic properties. [, ]
Q4: How does this compound contribute to the synthesis of complex molecules?
A4: this compound serves as a valuable precursor in synthesizing structurally diverse compounds. For example, employing bis(trifluoroacetoxy)iodobenzene, PhI(OCOCF3)2, with this compound facilitates the synthesis of 1-pyrroline. This transformation leverages a Hofmann rearrangement of the amide followed by in situ ring expansion of the intermediate cyclobutylamine. This approach further allows for creating more complex structures like 2,3-dihydro-1H-pyrrolo[2,1-a]isoquinolinium salts. []
Q5: What makes this compound a suitable substrate for palladium-catalyzed C-H activation reactions?
A5: this compound, when paired with directing groups like 8-aminoquinoline, readily undergoes palladium-catalyzed direct bis-arylation. This reaction allows for the diastereoselective introduction of aryl groups at the methylene C(sp3)-H bonds, generating trisubstituted this compound derivatives with an all-cis stereochemistry. The stereochemical outcome is often confirmed using X-ray crystallography of representative products. [, ]
Q6: Are there studies investigating this compound derivatives for potential antibacterial activity?
A6: Yes, recent research has focused on identifying novel antibacterial agents targeting the riboflavin synthesis pathway in multi-drug resistant Streptococcus pneumoniae (MDRSp). One promising approach involves inhibiting 3,4-dihydroxy-2-butanone-4-phosphate (SpDHBP) synthase, an enzyme crucial for riboflavin synthesis in MDRSp. Compounds like N-(3-acetamido-4-methyl-phenyl)-3-(4-fluorophenyl)-1H-pyrazole-4-carboxamide and N-[(1S)-2-[2-fluoro-5-(2-furyl)anilino]-1-methyl-2-oxo-ethyl]this compound have emerged as potential SpDHBP synthase inhibitors. These compounds exhibit promising pIC50 values, surpassing those of several existing antibiotics. Further research is necessary to explore their efficacy and safety profiles. []
Q7: What insights do we have into the conformational dynamics of melatonin receptors bound to this compound-based ligands?
A7: Bioluminescence resonance energy transfer (BRET) studies using this compound derivatives have shed light on the conformational changes that occur within MT1/MT2 melatonin receptor heterodimers upon ligand binding. These studies revealed that ligands can induce distinct conformational changes in the heterodimer compared to MT2 homodimers, suggesting potential for developing heterodimer-selective compounds. Moreover, these studies helped identify ligands demonstrating selectivity for the MT1/MT2 heterodimer over the MT2 homodimer, further underscoring the value of such investigations in understanding receptor pharmacology. []
Disclaimer and Information on In-Vitro Research Products
Please be aware that all articles and product information presented on BenchChem are intended solely for informational purposes. The products available for purchase on BenchChem are specifically designed for in-vitro studies, which are conducted outside of living organisms. In-vitro studies, derived from the Latin term "in glass," involve experiments performed in controlled laboratory settings using cells or tissues. It is important to note that these products are not categorized as medicines or drugs, and they have not received approval from the FDA for the prevention, treatment, or cure of any medical condition, ailment, or disease. We must emphasize that any form of bodily introduction of these products into humans or animals is strictly prohibited by law. It is essential to adhere to these guidelines to ensure compliance with legal and ethical standards in research and experimentation.