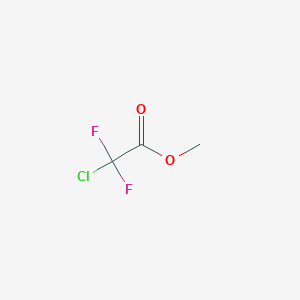
Methyl chlorodifluoroacetate
Overview
Description
Methyl chlorodifluoroacetate (MCDF) is an organic compound with the molecular formula CHF2CO2CH3. It is a colorless, volatile liquid that is used as a reagent in organic synthesis and for the preparation of fluorinated compounds. MCDF has a wide range of applications in scientific research and laboratory experiments. In
Scientific Research Applications
Trifluoromethylation of Saturated Organic Halides : Methyl chlorodifluoroacetate is used for trifluoromethylation of saturated organic halides, providing a method to create trifluoromethyl derivatives. This is important in synthetic chemistry for modifying organic compounds (Qing‐Yun Chen & Jian-Xing Duan, 1993).
Generation of Trifluoromethylcopper : It serves in the generation of trifluoromethyl copper reagent, important in organic synthesis for producing trifluoromethyl aromatic compounds (J. Macneil & D. Burton, 1991).
Trifluoromethylating Agent : Used as a convenient trifluoromethylating agent, facilitating the production of trifluoromethyl derivatives, which are valuable in various synthetic applications (Qing‐Yun Chen, D. Su & Jian-Xing Duan, 1991).
Synthesis of Anti-Infective Agents : Plays a key role in the synthesis of methyl 6-chloro-5-(trifluoromethyl)nicotinate, an intermediate in creating novel anti-infective agents (J. Mulder et al., 2013).
Atmospheric Sink Study : Investigated for its role in atmospheric chemistry, particularly its reaction with chlorine atoms, contributing to our understanding of atmospheric processes and pollutant behavior (M. B. Blanco et al., 2016).
Esterification Processes : Used in esterification reactions, specifically in the synthesis of this compound from 1,2-dichloro-1,1,2-trifluoro-2-methoxyethane, showcasing its utility in organic synthesis (H. Quan et al., 2001).
Herbicide Research : Although not directly about this compound, this study on selective weed-killers highlights the broader context of research in chemical synthesis and application in agriculture (G. E. Blackman, 1945).
Difluorocyclopropanes Synthesis : Useful in the synthesis of gem-difluorocyclopropanes, indicating its importance in creating specialized organic compounds (G. A. Wheaton & D. Burton, 1977).
Spectroscopy Studies : Analyzed for its microwave spectrum, contributing to the understanding of molecular structures and dynamics (B. Long et al., 2011).
Coupling Reactions in Organic Synthesis : Utilized in coupling reactions with carbonyl compounds, demonstrating its value in creating complex organic molecules (T. Ishihara et al., 1991).
Safety and Hazards
Future Directions
Future research directions should focus on difluoromethylation that makes use of inexpensive reagents, feedstock chemicals, and operationally simple procedures . Furthermore, there are still gaps in the area of C(sp 2 )–CF 2 H bond formation such as meta -selective C–H difluoromethylation of (hetero)arenes or decarboxylative .
Mechanism of Action
Target of Action
Methyl chlorodifluoroacetate is a trifluoromethylating agent
Mode of Action
This can alter the properties of the target molecule, such as its reactivity, stability, and polarity .
Biochemical Pathways
It has been used in the preparation of 2,2-difluoro-3-hydroxyesters and gem-difluorocyclopropane , indicating its role in the synthesis of these compounds.
Result of Action
The results of this compound’s action are primarily seen in its role as a trifluoromethylating agent. It can introduce a trifluoromethyl group into a variety of organic compounds, altering their properties and potentially their biological activity .
properties
IUPAC Name |
methyl 2-chloro-2,2-difluoroacetate | |
---|---|---|
Source | PubChem | |
URL | https://pubchem.ncbi.nlm.nih.gov | |
Description | Data deposited in or computed by PubChem | |
InChI |
InChI=1S/C3H3ClF2O2/c1-8-2(7)3(4,5)6/h1H3 | |
Source | PubChem | |
URL | https://pubchem.ncbi.nlm.nih.gov | |
Description | Data deposited in or computed by PubChem | |
InChI Key |
AWUPLMYXZJKHEG-UHFFFAOYSA-N | |
Source | PubChem | |
URL | https://pubchem.ncbi.nlm.nih.gov | |
Description | Data deposited in or computed by PubChem | |
Canonical SMILES |
COC(=O)C(F)(F)Cl | |
Source | PubChem | |
URL | https://pubchem.ncbi.nlm.nih.gov | |
Description | Data deposited in or computed by PubChem | |
Molecular Formula |
C3H3ClF2O2 | |
Source | PubChem | |
URL | https://pubchem.ncbi.nlm.nih.gov | |
Description | Data deposited in or computed by PubChem | |
DSSTOX Substance ID |
DTXSID5061742 | |
Record name | Methyl chlorodifluoroacetate | |
Source | EPA DSSTox | |
URL | https://comptox.epa.gov/dashboard/DTXSID5061742 | |
Description | DSSTox provides a high quality public chemistry resource for supporting improved predictive toxicology. | |
Molecular Weight |
144.50 g/mol | |
Source | PubChem | |
URL | https://pubchem.ncbi.nlm.nih.gov | |
Description | Data deposited in or computed by PubChem | |
CAS RN |
1514-87-0 | |
Record name | Methyl 2-chloro-2,2-difluoroacetate | |
Source | CAS Common Chemistry | |
URL | https://commonchemistry.cas.org/detail?cas_rn=1514-87-0 | |
Description | CAS Common Chemistry is an open community resource for accessing chemical information. Nearly 500,000 chemical substances from CAS REGISTRY cover areas of community interest, including common and frequently regulated chemicals, and those relevant to high school and undergraduate chemistry classes. This chemical information, curated by our expert scientists, is provided in alignment with our mission as a division of the American Chemical Society. | |
Explanation | The data from CAS Common Chemistry is provided under a CC-BY-NC 4.0 license, unless otherwise stated. | |
Record name | Acetic acid, 2-chloro-2,2-difluoro-, methyl ester | |
Source | ChemIDplus | |
URL | https://pubchem.ncbi.nlm.nih.gov/substance/?source=chemidplus&sourceid=0001514870 | |
Description | ChemIDplus is a free, web search system that provides access to the structure and nomenclature authority files used for the identification of chemical substances cited in National Library of Medicine (NLM) databases, including the TOXNET system. | |
Record name | Acetic acid, 2-chloro-2,2-difluoro-, methyl ester | |
Source | EPA Chemicals under the TSCA | |
URL | https://www.epa.gov/chemicals-under-tsca | |
Description | EPA Chemicals under the Toxic Substances Control Act (TSCA) collection contains information on chemicals and their regulations under TSCA, including non-confidential content from the TSCA Chemical Substance Inventory and Chemical Data Reporting. | |
Record name | Methyl chlorodifluoroacetate | |
Source | EPA DSSTox | |
URL | https://comptox.epa.gov/dashboard/DTXSID5061742 | |
Description | DSSTox provides a high quality public chemistry resource for supporting improved predictive toxicology. | |
Record name | Methyl chlorodifluoroacetate | |
Source | European Chemicals Agency (ECHA) | |
URL | https://echa.europa.eu/substance-information/-/substanceinfo/100.014.686 | |
Description | The European Chemicals Agency (ECHA) is an agency of the European Union which is the driving force among regulatory authorities in implementing the EU's groundbreaking chemicals legislation for the benefit of human health and the environment as well as for innovation and competitiveness. | |
Explanation | Use of the information, documents and data from the ECHA website is subject to the terms and conditions of this Legal Notice, and subject to other binding limitations provided for under applicable law, the information, documents and data made available on the ECHA website may be reproduced, distributed and/or used, totally or in part, for non-commercial purposes provided that ECHA is acknowledged as the source: "Source: European Chemicals Agency, http://echa.europa.eu/". Such acknowledgement must be included in each copy of the material. ECHA permits and encourages organisations and individuals to create links to the ECHA website under the following cumulative conditions: Links can only be made to webpages that provide a link to the Legal Notice page. | |
Retrosynthesis Analysis
AI-Powered Synthesis Planning: Our tool employs the Template_relevance Pistachio, Template_relevance Bkms_metabolic, Template_relevance Pistachio_ringbreaker, Template_relevance Reaxys, Template_relevance Reaxys_biocatalysis model, leveraging a vast database of chemical reactions to predict feasible synthetic routes.
One-Step Synthesis Focus: Specifically designed for one-step synthesis, it provides concise and direct routes for your target compounds, streamlining the synthesis process.
Accurate Predictions: Utilizing the extensive PISTACHIO, BKMS_METABOLIC, PISTACHIO_RINGBREAKER, REAXYS, REAXYS_BIOCATALYSIS database, our tool offers high-accuracy predictions, reflecting the latest in chemical research and data.
Strategy Settings
Precursor scoring | Relevance Heuristic |
---|---|
Min. plausibility | 0.01 |
Model | Template_relevance |
Template Set | Pistachio/Bkms_metabolic/Pistachio_ringbreaker/Reaxys/Reaxys_biocatalysis |
Top-N result to add to graph | 6 |
Feasible Synthetic Routes
Q & A
Q1: What is the atmospheric fate of methyl chlorodifluoroacetate?
A: [] this compound (MCDFA) reacts with chlorine atoms in the atmosphere. This reaction leads to the formation of chlorofluoroacetic acid (CF2ClC(O)OH) with a yield of approximately 34%. The primary pathway for this formation involves the reaction of alkoxy radicals (formed after H-atom abstraction by Cl) with oxygen (O2).
Q2: How does the atmospheric reactivity of this compound compare to ethyl chlorodifluoroacetate?
A: [] While both MCDFA and ethyl chlorodifluoroacetate (ECDFA) react with chlorine atoms in the atmosphere, the rate coefficient for ECDFA is significantly higher. This means ECDFA is removed from the atmosphere more quickly than MCDFA. Interestingly, the yield of chlorofluoroacetic acid is also significantly higher for ECDFA (86%) compared to MCDFA (34%). This difference is attributed to the dominant degradation pathway for each compound: O2 reaction for MCDFA and α-ester rearrangement for ECDFA.
Q3: Can this compound be used to introduce trifluoromethyl groups into molecules?
A: [, , ] Yes, this compound can act as a convenient trifluoromethylating agent in organic synthesis. For example, it can be used in the presence of potassium fluoride (KF) and copper iodide (CuI) to introduce a trifluoromethyl group onto an aryl iodide, as demonstrated in the synthesis of methyl 6-chloro-5-(trifluoromethyl)nicotinate.
Q4: Are there alternative methods for generating difluorocarbene from this compound?
A: [, ] Yes, besides using this compound as a trifluoromethylating agent, it can also be used as a precursor for generating difluorocarbene. One method involves decomposition by alkali metal halides. Another approach utilizes an electrochemical, nickel-catalyzed Reformatsky reaction with MCDFA.
Q5: What is known about the structure of this compound?
A: [] The microwave spectrum of MCDFA has been studied, providing information about its structure. The study revealed details about the methyl internal rotation and chlorine nuclear electric quadrupole coupling within the MCDFA molecule.
Q6: Can this compound be used to make polymers?
A: [] Yes, chlorodifluoroacetaldehyde, a precursor to MCDFA, can be polymerized to create polychlorodifluoroacetaldehyde. This polymer can exist in both crystalline and amorphous forms, depending on the polymerization conditions. The thermal stability of the polymer can be improved by end-capping with acetylation.
Q7: Can this compound be used in the synthesis of pharmaceutically relevant compounds?
A: [] Yes, MCDFA can be employed in multistep synthesis to create compounds with potential pharmaceutical applications. For example, it is utilized in the synthesis of 3-(difluoromethoxy)-N-(3, 5-dichloropyridin-4-yl)-4-(cyclopropylmethoxy) benzamide, which may have potential use as a pharmaceutical agent.
Disclaimer and Information on In-Vitro Research Products
Please be aware that all articles and product information presented on BenchChem are intended solely for informational purposes. The products available for purchase on BenchChem are specifically designed for in-vitro studies, which are conducted outside of living organisms. In-vitro studies, derived from the Latin term "in glass," involve experiments performed in controlled laboratory settings using cells or tissues. It is important to note that these products are not categorized as medicines or drugs, and they have not received approval from the FDA for the prevention, treatment, or cure of any medical condition, ailment, or disease. We must emphasize that any form of bodily introduction of these products into humans or animals is strictly prohibited by law. It is essential to adhere to these guidelines to ensure compliance with legal and ethical standards in research and experimentation.