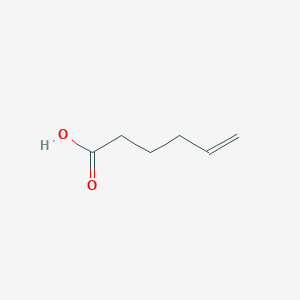
5-Hexenoic acid
Overview
Description
5-Hexenoic acid: is an organic compound with the molecular formula C6H10O2 It is a medium-chain fatty acid characterized by the presence of a double bond between the fifth and sixth carbon atoms in its structureHex-5-enoic acid . It is a colorless to light yellow liquid with a density of 0.961 g/mL at 25°C and a boiling point of 105°C at 15 mmHg .
Mechanism of Action
Target of Action
5-Hexenoic acid, also known as γ-Vinyl-GABA or Vigabatrin , is a structural analogue of gamma-aminobutyric acid (GABA), the main inhibitory neurotransmitter in the central nervous system . Its primary target is GABA transaminase, the enzyme responsible for the catabolism of GABA .
Mode of Action
This compound acts as an irreversible mechanism-based inhibitor of GABA transaminase . By inhibiting this enzyme, it increases the levels of GABA in the brain . This potentiation of GABAergic neurotransmission is a crucial mechanism through which antiepileptic agents may combat the pathologic excitatory neurotransmission seen in epilepsy .
Biochemical Pathways
The increase in GABA levels in the brain due to the inhibition of GABA transaminase by this compound affects the GABAergic neurotransmission pathway . This leads to an increase in inhibitory signals in the central nervous system, which can help to control seizures .
Pharmacokinetics
It has a bioavailability of 80-90% .
Result of Action
The increase in GABA levels in the brain due to the action of this compound can lead to a decrease in the frequency and severity of seizures in individuals with epilepsy . It should be noted that the use of this compound can be associated with some side effects, including permanent vision loss .
Preparation Methods
Synthetic Routes and Reaction Conditions:
From 5-Hexen-1-ol: One common method for synthesizing 5-Hexenoic acid involves the oxidation of 5-Hexen-1-ol.
From 5-Hexenyl Bromide: Another method involves the hydrolysis of 5-Hexenyl bromide in the presence of a strong base like sodium hydroxide (NaOH) followed by acidification.
Industrial Production Methods:
Catalytic Oxidation: Industrially, this compound can be produced via catalytic oxidation of 5-Hexen-1-ol using catalysts such as platinum or palladium supported on carbon.
Grignard Reaction: Another industrial method involves the Grignard reaction where 5-Hexenyl magnesium bromide reacts with carbon dioxide (CO2) to form this compound.
Chemical Reactions Analysis
Types of Reactions:
Oxidation: 5-Hexenoic acid can undergo oxidation reactions to form various products.
Substitution: The double bond in this compound allows for various substitution reactions.
Common Reagents and Conditions:
Oxidizing Agents: Potassium permanganate (KMnO4), chromium trioxide (CrO3).
Reducing Agents: Lithium aluminum hydride (LiAlH4).
Halogenating Agents: Bromine (Br2).
Major Products Formed:
Oxidation: this compound derivatives.
Reduction: 5-Hexen-1-ol.
Substitution: 5-Bromohexanoic acid.
Scientific Research Applications
Chemistry:
Synthesis Intermediate: 5-Hexenoic acid is used as an intermediate in the synthesis of various organic compounds, including iodolactone derivatives and fluorine-containing δ-valerolactones.
Biology and Medicine:
Pharmaceuticals: this compound derivatives are explored for their potential use in pharmaceuticals, particularly in the synthesis of compounds with biological activity.
Industry:
Comparison with Similar Compounds
4-Pentenoic acid: Similar structure but with a double bond between the fourth and fifth carbon atoms.
6-Heptenoic acid: Similar structure but with a longer carbon chain.
5-Hexynoic acid: Similar structure but with a triple bond instead of a double bond.
Uniqueness:
Double Bond Position: The position of the double bond in 5-Hexenoic acid makes it unique compared to other similar compounds. This influences its reactivity and the types of reactions it can undergo.
Properties
IUPAC Name |
hex-5-enoic acid | |
---|---|---|
Source | PubChem | |
URL | https://pubchem.ncbi.nlm.nih.gov | |
Description | Data deposited in or computed by PubChem | |
InChI |
InChI=1S/C6H10O2/c1-2-3-4-5-6(7)8/h2H,1,3-5H2,(H,7,8) | |
Source | PubChem | |
URL | https://pubchem.ncbi.nlm.nih.gov | |
Description | Data deposited in or computed by PubChem | |
InChI Key |
XUDOZULIAWNMIU-UHFFFAOYSA-N | |
Source | PubChem | |
URL | https://pubchem.ncbi.nlm.nih.gov | |
Description | Data deposited in or computed by PubChem | |
Canonical SMILES |
C=CCCCC(=O)O | |
Source | PubChem | |
URL | https://pubchem.ncbi.nlm.nih.gov | |
Description | Data deposited in or computed by PubChem | |
Molecular Formula |
C6H10O2 | |
Source | PubChem | |
URL | https://pubchem.ncbi.nlm.nih.gov | |
Description | Data deposited in or computed by PubChem | |
DSSTOX Substance ID |
DTXSID30166305 | |
Record name | 5-Hexenoic acid | |
Source | EPA DSSTox | |
URL | https://comptox.epa.gov/dashboard/DTXSID30166305 | |
Description | DSSTox provides a high quality public chemistry resource for supporting improved predictive toxicology. | |
Molecular Weight |
114.14 g/mol | |
Source | PubChem | |
URL | https://pubchem.ncbi.nlm.nih.gov | |
Description | Data deposited in or computed by PubChem | |
CAS No. |
1577-22-6 | |
Record name | 5-Hexenoic acid | |
Source | ChemIDplus | |
URL | https://pubchem.ncbi.nlm.nih.gov/substance/?source=chemidplus&sourceid=0001577226 | |
Description | ChemIDplus is a free, web search system that provides access to the structure and nomenclature authority files used for the identification of chemical substances cited in National Library of Medicine (NLM) databases, including the TOXNET system. | |
Record name | 5-Hexenoic acid | |
Source | EPA DSSTox | |
URL | https://comptox.epa.gov/dashboard/DTXSID30166305 | |
Description | DSSTox provides a high quality public chemistry resource for supporting improved predictive toxicology. | |
Record name | 5-Hexenoic Acid | |
Source | European Chemicals Agency (ECHA) | |
URL | https://echa.europa.eu/information-on-chemicals | |
Description | The European Chemicals Agency (ECHA) is an agency of the European Union which is the driving force among regulatory authorities in implementing the EU's groundbreaking chemicals legislation for the benefit of human health and the environment as well as for innovation and competitiveness. | |
Explanation | Use of the information, documents and data from the ECHA website is subject to the terms and conditions of this Legal Notice, and subject to other binding limitations provided for under applicable law, the information, documents and data made available on the ECHA website may be reproduced, distributed and/or used, totally or in part, for non-commercial purposes provided that ECHA is acknowledged as the source: "Source: European Chemicals Agency, http://echa.europa.eu/". Such acknowledgement must be included in each copy of the material. ECHA permits and encourages organisations and individuals to create links to the ECHA website under the following cumulative conditions: Links can only be made to webpages that provide a link to the Legal Notice page. | |
Record name | 5-HEXENOIC ACID | |
Source | FDA Global Substance Registration System (GSRS) | |
URL | https://gsrs.ncats.nih.gov/ginas/app/beta/substances/5HL6OL352W | |
Description | The FDA Global Substance Registration System (GSRS) enables the efficient and accurate exchange of information on what substances are in regulated products. Instead of relying on names, which vary across regulatory domains, countries, and regions, the GSRS knowledge base makes it possible for substances to be defined by standardized, scientific descriptions. | |
Explanation | Unless otherwise noted, the contents of the FDA website (www.fda.gov), both text and graphics, are not copyrighted. They are in the public domain and may be republished, reprinted and otherwise used freely by anyone without the need to obtain permission from FDA. Credit to the U.S. Food and Drug Administration as the source is appreciated but not required. | |
Retrosynthesis Analysis
AI-Powered Synthesis Planning: Our tool employs the Template_relevance Pistachio, Template_relevance Bkms_metabolic, Template_relevance Pistachio_ringbreaker, Template_relevance Reaxys, Template_relevance Reaxys_biocatalysis model, leveraging a vast database of chemical reactions to predict feasible synthetic routes.
One-Step Synthesis Focus: Specifically designed for one-step synthesis, it provides concise and direct routes for your target compounds, streamlining the synthesis process.
Accurate Predictions: Utilizing the extensive PISTACHIO, BKMS_METABOLIC, PISTACHIO_RINGBREAKER, REAXYS, REAXYS_BIOCATALYSIS database, our tool offers high-accuracy predictions, reflecting the latest in chemical research and data.
Strategy Settings
Precursor scoring | Relevance Heuristic |
---|---|
Min. plausibility | 0.01 |
Model | Template_relevance |
Template Set | Pistachio/Bkms_metabolic/Pistachio_ringbreaker/Reaxys/Reaxys_biocatalysis |
Top-N result to add to graph | 6 |
Feasible Synthetic Routes
Disclaimer and Information on In-Vitro Research Products
Please be aware that all articles and product information presented on BenchChem are intended solely for informational purposes. The products available for purchase on BenchChem are specifically designed for in-vitro studies, which are conducted outside of living organisms. In-vitro studies, derived from the Latin term "in glass," involve experiments performed in controlled laboratory settings using cells or tissues. It is important to note that these products are not categorized as medicines or drugs, and they have not received approval from the FDA for the prevention, treatment, or cure of any medical condition, ailment, or disease. We must emphasize that any form of bodily introduction of these products into humans or animals is strictly prohibited by law. It is essential to adhere to these guidelines to ensure compliance with legal and ethical standards in research and experimentation.