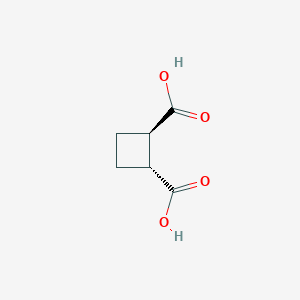
trans-Cyclobutane-1,2-dicarboxylic acid
Overview
Description
trans-Cyclobutane-1,2-dicarboxylic acid (CAS No. 1124-13-6) is a cyclic aliphatic dicarboxylic acid with a four-membered cyclobutane ring and two carboxyl groups in a trans-configuration. It is a white crystalline solid with a molecular formula of C₆H₈O₄ and a molecular weight of 144.13 g/mol . Key physicochemical properties include:
- Melting point: 125–131 °C
- Boiling point: 140 °C (at 2 Torr)
- Density: 1.509 g/cm³
- pKa values: 3.79 (pK₁) and 5.61 (pK₂) at 25 °C .
This compound is widely used as an intermediate in polymer synthesis (e.g., polyimides) and pharmaceuticals (e.g., metformin) due to its rigid yet reactive structure . Industrially, it is synthesized via hydrolysis of the anhydride formed from cyclobutanol and maleic anhydride under mild conditions .
Preparation Methods
Synthesis via Cyclobutane-1,2-Dicyanide Hydrolysis
The most well-documented method for synthesizing trans-cyclobutane-1,2-dicarboxylic acid involves the hydrolysis of trans-cyclobutane-1,2-dicyanide. This two-step process begins with the preparation of the dicyanide precursor, followed by its controlled hydrolysis under acidic conditions.
Preparation of trans-Cyclobutane-1,2-Dicyanide
trans-Cyclobutane-1,2-dicyanide is synthesized via the dimerization of acrylonitrile under photochemical or thermal conditions, forming a mixture of cis and trans isomers . The reaction proceeds via a [2+2] cycloaddition mechanism, yielding approximately equal proportions of both isomers. Separation of the trans isomer is achieved through fractional vacuum distillation, leveraging differences in boiling points due to steric and dipole interactions .
Hydrolysis with Sulfuric Acid Monohydrate
The isolated trans-dicyanide undergoes hydrolysis using sulfuric acid monohydrate (H₂SO₄·H₂O) under carefully controlled conditions. Key parameters include:
-
Temperature : 40–125°C (optimized at 90°C for industrial scalability) .
-
Reaction Time : 15 minutes to 4 hours, inversely proportional to temperature .
-
Acid-to-Dicyanide Ratio : A molar ratio of 2:1 ensures complete adduct formation .
The reaction mechanism involves the formation of an intermediate adduct between the dicyanide and sulfuric acid, followed by nucleophilic attack of water on the nitrile groups. This stepwise hydrolysis preserves the trans configuration, avoiding isomerization observed in hydrochloric acid-mediated reactions .
Example Protocol:
-
Adduct Formation : A mixture of 233 g trans-dicyanide and 572 g sulfuric acid monohydrate is stirred at 90°C for 15 minutes.
-
Hydrolysis : Ice-cold water (200 mL) is rapidly added, inducing exothermic hydrolysis (temperature peaks at 140°C).
-
Isolation : The product precipitates upon cooling and is purified via recrystallization from water, yielding 287 g (91%) of this compound .
Critical Reaction Parameters and Optimization
Temperature and Time Dependence
Elevated temperatures accelerate adduct formation but risk side reactions such as ring-opening or isomerization. At 90°C, the reaction reaches completion within 15 minutes, whereas at 40°C, 4 hours are required . Industrial processes favor higher temperatures for throughput, provided cooling is implemented post-reaction to mitigate degradation.
Acid Selection and Concentration
Sulfuric acid monohydrate is preferred over anhydrous sulfuric acid or hydrochloric acid due to its dual role as a catalyst and water source. Hydrochloric acid induces partial racemization, producing mixed cis/trans products, whereas sulfuric acid preserves stereochemical integrity .
Solvent and Workup Considerations
The reaction is conducted solvent-free to maximize efficiency. Post-hydrolysis, the trans isomer crystallizes directly from the aqueous phase, simplifying isolation. By contrast, the cis isomer requires extraction with water-immiscible solvents (e.g., diethyl ether), adding complexity to its purification .
Industrial Scalability and Challenges
Continuous Flow Reactors
Modern production facilities employ continuous flow systems to enhance yield and consistency. Key advantages include:
-
Precise temperature control via jacketed reactors.
-
Reduced batch-to-batch variability.
Byproduct Management
The primary byproduct, ammonium sulfate, is removed via filtration and repurposed as fertilizer. Trace impurities (e.g., unreacted dicyanide) are eliminated through activated carbon treatment, ensuring >99% purity in the final product .
Comparative Analysis of Synthetic Routes
*Alternative methods include electrochemical carboxylation and cycloaddition of maleic anhydride derivatives, which are less commonly employed due to lower efficiency .
Characterization and Quality Control
The final product is validated using:
Chemical Reactions Analysis
Types of Reactions:
Oxidation: trans-Cyclobutane-1,2-dicarboxylic acid can undergo oxidation reactions, typically using strong oxidizing agents like potassium permanganate or chromium trioxide, leading to the formation of diketone derivatives.
Reduction: Reduction reactions can convert the carboxylic acid groups into alcohols or aldehydes, using reagents such as lithium aluminum hydride or borane.
Substitution: The compound can participate in nucleophilic substitution reactions, where the carboxylic acid groups are replaced by other functional groups like esters or amides, using reagents like thionyl chloride or carbodiimides.
Common Reagents and Conditions:
Oxidation: Potassium permanganate (KMnO4), chromium trioxide (CrO3)
Reduction: Lithium aluminum hydride (LiAlH4), borane (BH3)
Substitution: Thionyl chloride (SOCl2), carbodiimides (e.g., DCC)
Major Products:
Oxidation: Diketone derivatives
Reduction: Alcohols, aldehydes
Substitution: Esters, amides
Scientific Research Applications
Polymer Chemistry
Overview : CBDA serves as a crucial building block in the synthesis of various polymers. Its unique structure enhances the properties of these materials, particularly in terms of flexibility and thermal stability.
Applications :
- Specialty Plastics : CBDA is utilized in creating specialty plastics that require enhanced mechanical properties.
- Thermoplastics and Thermosets : Recent studies have shown that CBDA can be incorporated into both thermoplastics and thermosets, leading to materials with excellent mechanical performance and thermal stability .
Case Study : Research has demonstrated that CBDA derivatives can be used as cross-linkers in polymer synthesis, resulting in materials with improved durability and recyclability. For example, the incorporation of CBDA into polyester resins has been shown to enhance thermal properties significantly .
Pharmaceuticals
Overview : The structural characteristics of CBDA allow for the development of novel drug candidates. Its ability to interact with biological targets makes it a promising candidate for targeted therapies.
Applications :
- Drug Development : Researchers are exploring CBDA's potential in designing compounds that can effectively engage with specific biological receptors.
- Antinociceptive Activities : Some derivatives of CBDA have shown promise in pain relief applications, indicating potential for therapeutic use .
Case Study : A study on the synthesis of CBDA derivatives revealed their effectiveness as internal donors in Ziegler-Natta catalysts for propylene polymerization, showcasing their utility beyond traditional pharmaceutical applications .
Food Industry
Overview : In the food sector, CBDA is used as an additive to enhance flavor and preserve food products.
Applications :
- Flavor Enhancer : CBDA can improve the taste profile of various food items.
- Preservative Properties : Its application helps extend the shelf life of products while adhering to safety standards.
Cosmetic Formulations
Overview : The cosmetic industry incorporates CBDA into formulations to enhance product performance.
Applications :
- Texture Improvement : CBDA contributes to better texture and stability in skincare products.
- Stability Enhancement : Its inclusion can lead to increased shelf life and efficacy of cosmetic formulations.
Environmental Applications
Overview : With growing concerns about plastic waste, CBDA is being researched for its role in developing biodegradable materials.
Applications :
- Biodegradable Plastics : Studies are ongoing to explore how CBDA can be integrated into biodegradable plastics to reduce environmental impact.
- Sustainable Practices : The use of CBDA in eco-friendly alternatives promotes sustainable practices within various industries .
Data Table: Summary of Applications
Application Area | Specific Uses | Benefits |
---|---|---|
Polymer Chemistry | Specialty plastics, thermoplastics | Enhanced flexibility, thermal stability |
Pharmaceuticals | Drug development | Targeted therapies, antinociceptive activities |
Food Industry | Flavor enhancer, preservative | Improved taste and shelf life |
Cosmetic Formulations | Texture improvement | Enhanced product performance |
Environmental Applications | Biodegradable plastics | Reduction of plastic waste |
Mechanism of Action
The mechanism by which trans-cyclobutane-1,2-dicarboxylic acid exerts its effects depends on its application. In drug development, it may act by binding to specific molecular targets, such as enzymes or receptors, thereby modulating their activity. The rigid cyclobutane ring provides a unique spatial arrangement that can enhance binding specificity and affinity. In polymer chemistry, the compound’s structure influences the physical properties of the resulting polymers, such as rigidity and thermal stability.
Comparison with Similar Compounds
Comparison with Structurally Similar Compounds
Cis-Cyclobutane-1,2-dicarboxylic Acid (CAS 1461-94-5)
The cis-isomer differs in the spatial arrangement of carboxyl groups, leading to distinct properties:
- Fragmentation patterns : Mass spectrometry distinguishes cis and trans isomers due to interactions between carboxyl groups during ionization .
- Thermodynamic stability : The trans isomer is generally more stable due to reduced steric hindrance between carboxyl groups .
- Applications : The cis isomer is less commonly used industrially but serves as a reference in conformational studies .
Cyclobutane-1,1-dicarboxylic Acid (CAS 5445-51-2)
This 1,1-dicarboxylate isomer has both carboxyl groups on the same carbon atom:
- Acidity : Higher acidity compared to 1,2-isomers due to proximity of electron-withdrawing groups .
- Pharmaceutical relevance : Used as a precursor for carboplatin impurities and other platinum-based drugs .
trans-Cyclobutane-1,3-dicarboxylic Acid (CAS 7439-33-0)
A positional isomer with carboxyl groups at the 1 and 3 positions:
- Ring strain : Reduced steric strain compared to 1,2-isomers, enhancing solubility in polar solvents .
- Reactivity : Prefers forming intramolecular hydrogen bonds, altering its reactivity in polymerization .
Cyclopropane and Cyclohexane Analogs
- trans-3-Methylenecyclopropane-1,2-dicarboxylic acid : Smaller cyclopropane ring increases ring strain, making it more reactive in Diels-Alder reactions .
- 4-Cyclohexene-1,2-dicarboxylic acid : Used in epoxy resins; its larger ring reduces rigidity but improves flexibility in polymer matrices .
Data Table: Key Properties of Cyclobutane Dicarboxylates
Compound | CAS No. | Configuration | Melting Point (°C) | pKa (25°C) | Key Applications |
---|---|---|---|---|---|
trans-Cyclobutane-1,2-dicarboxylic acid | 1124-13-6 | trans | 125–131 | 3.79, 5.61 | Polymers, pharmaceuticals |
cis-Cyclobutane-1,2-dicarboxylic acid | 1461-94-5 | cis | Not reported | Not reported | Conformational studies |
Cyclobutane-1,1-dicarboxylic acid | 5445-51-2 | 1,1 | 185–190 | 2.1, 4.7 (est.) | Drug intermediates |
trans-Cyclobutane-1,3-dicarboxylic acid | 7439-33-0 | trans | Not reported | Not reported | Specialty polymers |
Biological Activity
trans-Cyclobutane-1,2-dicarboxylic acid (trans-CBDA) is a cyclic dicarboxylic acid characterized by its four-membered cyclobutane ring and two carboxylic acid functional groups. This compound has garnered attention for its unique structural properties and potential biological activities, making it a valuable subject of research in organic chemistry and materials science.
trans-CBDA has the molecular formula and a molecular weight of approximately 144.13 g/mol. The trans configuration of the carboxylic acid groups minimizes steric hindrance, contributing to the compound's stability and reactivity. The rigid structure allows for various chemical interactions, particularly as a precursor in synthesizing more complex organic molecules and polymers.
Biological Activity
Chelating Properties : One of the notable biological activities of trans-CBDA is its ability to act as a chelating agent. The carboxylic acid groups can bind to metal ions, which is significant in studying metal-ligand interactions that play essential roles in biological processes. For example, trans-CBDA may be explored for its potential applications in drug delivery systems or as a stabilizing agent in biochemical assays.
Polymerization Potential : The compound's structure allows it to participate in various polymerization techniques, leading to the development of novel materials with unique properties. These materials could have applications in drug delivery, tissue engineering, and other biomedical fields due to their biocompatibility and tailored mechanical properties .
Research Findings
Recent studies have highlighted several aspects of trans-CBDA's biological activity:
- Stability and Reactivity : Research indicates that trans-CBDA maintains stability under various conditions, including exposure to acids and bases. This stability is crucial for its application in creating durable materials .
- Thermal Properties : Thermogravimetric analysis (TGA) and differential scanning calorimetry (DSC) studies suggest that trans-CBDA exhibits significant thermal stability, making it suitable for high-temperature applications .
- Photochemical Behavior : Studies have shown that trans-CBDA can undergo photodimerization reactions, leading to new compounds with potentially enhanced biological activities. This property could be exploited in developing light-responsive materials .
Case Studies
- Metal Ion Binding Studies : A study investigated the binding affinity of trans-CBDA with various metal ions. The findings indicated that trans-CBDA effectively chelates ions such as Cu²⁺ and Zn²⁺, suggesting potential applications in bioremediation and environmental chemistry.
- Polymer Synthesis : Researchers synthesized poly(α-truxillates) from trans-CBDA through condensation reactions with diols. These polymers exhibited promising mechanical properties and biocompatibility, indicating their potential use in medical applications such as scaffolds for tissue engineering .
- Thermal Stability Analysis : A comprehensive analysis using TGA revealed that trans-CBDA retains structural integrity up to temperatures exceeding 200 °C, which is advantageous for applications requiring thermal resistance .
Comparative Analysis
The following table summarizes the unique features of trans-CBDA compared to structurally similar compounds:
Compound Name | Molecular Formula | Unique Features |
---|---|---|
This compound | C₆H₈O₄ | Four-membered ring; stable under various conditions |
Cyclopentane-1,2-dicarboxylic acid | C₅H₈O₄ | Five-membered ring; higher strain energy |
cis-Cyclobutane-1,2-dicarboxylic acid | C₆H₈O₄ | Different stereochemistry; potentially different reactivity |
Maleic acid | C₄H₄O₄ | Fewer carbon atoms; unsaturated dicarboxylic acid |
Q & A
Basic Research Questions
Q. What are the standard synthetic protocols for trans-cyclobutane-1,2-dicarboxylic acid?
The compound is synthesized via a two-step process: (1) reaction of cyclobutanol with maleic anhydride under mild conditions (ambient temperature, non-polar solvents), followed by (2) hydrolysis of the intermediate anhydride using aqueous acid or base. This method offers high efficiency and scalability with readily available reagents .
Q. How can cis and trans isomers of cyclobutane-1,2-dicarboxylic acid be distinguished experimentally?
Mass spectrometry (MS) is a key method. The cis isomer exhibits distinct fragmentation patterns due to steric interactions between carboxyl groups, leading to unique ion peaks. In contrast, the trans isomer’s carboxyl groups are spatially separated, resulting in simpler fragmentation .
Q. What physicochemical properties are critical for its reactivity in organic synthesis?
Key properties include:
- Melting point: 125–131°C (indicates purity and stability).
- pKa values: 3.79 (first dissociation) and 5.61 (second dissociation), influencing solubility and acid-base reactivity.
- Density: 1.509 g/cm³ (relevant for solvent selection in reactions) .
Q. What spectroscopic techniques are used to confirm its structural integrity?
- NMR : and NMR identify proton environments and confirm trans-configuration via coupling constants.
- IR Spectroscopy : Carboxyl C=O stretching vibrations (~1700 cm⁻¹) and O-H stretches (~2500–3000 cm⁻¹) validate functional groups .
Advanced Research Questions
Q. How does the trans-configuration influence its application in polymer chemistry?
The trans-arranged carboxyl groups enable bifunctional crosslinking in polymers, enhancing thermal stability and mechanical strength. For example, derivatives of cyclobutane dicarboxylic acids are used in recyclable thermosets, where the trans-configuration facilitates controlled cleavage at high temperatures (e.g., 200–250°C) .
Q. What computational models predict the thermodynamic stability of the trans-isomer compared to cis?
Density Functional Theory (DFT) calculations reveal that the trans-isomer is ~15 kJ/mol more stable due to reduced ring strain and minimized steric hindrance between carboxyl groups. Experimental validation via calorimetry aligns with these predictions .
Q. How can discrepancies between experimental and computational pKa values be resolved?
Discrepancies often arise from solvent effects (e.g., dielectric constant) and ionic strength. Potentiometric titration in controlled conditions (e.g., 0.1 M KCl) or COSMO-RS solvation models improve alignment between observed and predicted pKa .
Q. What role does it play in synthesizing pharmaceutical intermediates like metformin analogs?
The carboxyl groups participate in hydrogen bonding and coordination with metal catalysts during heterocycle formation. For example, it serves as a precursor in dihydrofolate reductase inhibitors, where stereochemistry impacts drug bioavailability .
Q. What challenges arise in characterizing its solid-state polymorphism?
Polymorphs can form due to flexible cyclobutane rings. X-ray diffraction (XRD) and differential scanning calorimetry (DSC) are essential to identify crystalline phases. For instance, DSC thermograms show distinct endothermic peaks for different polymorphs .
Q. Can this compound enable recyclable materials?
Derivatives like cis-3,4-diphenylcyclobutane-1,2-dicarboxylic acid (CBDA-4) form thermosets with built-in thermocleavable bonds. Heating above 220°C breaks the cyclobutane ring, enabling depolymerization and reuse—a strategy applicable to trans-isomers with tailored substituents .
Properties
IUPAC Name |
(1R,2R)-cyclobutane-1,2-dicarboxylic acid | |
---|---|---|
Source | PubChem | |
URL | https://pubchem.ncbi.nlm.nih.gov | |
Description | Data deposited in or computed by PubChem | |
InChI |
InChI=1S/C6H8O4/c7-5(8)3-1-2-4(3)6(9)10/h3-4H,1-2H2,(H,7,8)(H,9,10)/t3-,4-/m1/s1 | |
Source | PubChem | |
URL | https://pubchem.ncbi.nlm.nih.gov | |
Description | Data deposited in or computed by PubChem | |
InChI Key |
SUSAGCZZQKACKE-QWWZWVQMSA-N | |
Source | PubChem | |
URL | https://pubchem.ncbi.nlm.nih.gov | |
Description | Data deposited in or computed by PubChem | |
Canonical SMILES |
C1CC(C1C(=O)O)C(=O)O | |
Source | PubChem | |
URL | https://pubchem.ncbi.nlm.nih.gov | |
Description | Data deposited in or computed by PubChem | |
Isomeric SMILES |
C1C[C@H]([C@@H]1C(=O)O)C(=O)O | |
Source | PubChem | |
URL | https://pubchem.ncbi.nlm.nih.gov | |
Description | Data deposited in or computed by PubChem | |
Molecular Formula |
C6H8O4 | |
Source | PubChem | |
URL | https://pubchem.ncbi.nlm.nih.gov | |
Description | Data deposited in or computed by PubChem | |
DSSTOX Substance ID |
DTXSID301031207 | |
Record name | (1R,2R)-Cyclobutane-1,2-dicarboxylic acid | |
Source | EPA DSSTox | |
URL | https://comptox.epa.gov/dashboard/DTXSID301031207 | |
Description | DSSTox provides a high quality public chemistry resource for supporting improved predictive toxicology. | |
Molecular Weight |
144.12 g/mol | |
Source | PubChem | |
URL | https://pubchem.ncbi.nlm.nih.gov | |
Description | Data deposited in or computed by PubChem | |
CAS No. |
1124-13-6 | |
Record name | (±)-trans-1,2-Cyclobutanedicarboxylic acid | |
Source | CAS Common Chemistry | |
URL | https://commonchemistry.cas.org/detail?cas_rn=1124-13-6 | |
Description | CAS Common Chemistry is an open community resource for accessing chemical information. Nearly 500,000 chemical substances from CAS REGISTRY cover areas of community interest, including common and frequently regulated chemicals, and those relevant to high school and undergraduate chemistry classes. This chemical information, curated by our expert scientists, is provided in alignment with our mission as a division of the American Chemical Society. | |
Explanation | The data from CAS Common Chemistry is provided under a CC-BY-NC 4.0 license, unless otherwise stated. | |
Record name | (1R,2R)-Cyclobutane-1,2-dicarboxylic acid | |
Source | EPA DSSTox | |
URL | https://comptox.epa.gov/dashboard/DTXSID301031207 | |
Description | DSSTox provides a high quality public chemistry resource for supporting improved predictive toxicology. | |
Record name | trans-cyclobutane-1,2-dicarboxylic acid | |
Source | European Chemicals Agency (ECHA) | |
URL | https://echa.europa.eu/substance-information/-/substanceinfo/100.013.085 | |
Description | The European Chemicals Agency (ECHA) is an agency of the European Union which is the driving force among regulatory authorities in implementing the EU's groundbreaking chemicals legislation for the benefit of human health and the environment as well as for innovation and competitiveness. | |
Explanation | Use of the information, documents and data from the ECHA website is subject to the terms and conditions of this Legal Notice, and subject to other binding limitations provided for under applicable law, the information, documents and data made available on the ECHA website may be reproduced, distributed and/or used, totally or in part, for non-commercial purposes provided that ECHA is acknowledged as the source: "Source: European Chemicals Agency, http://echa.europa.eu/". Such acknowledgement must be included in each copy of the material. ECHA permits and encourages organisations and individuals to create links to the ECHA website under the following cumulative conditions: Links can only be made to webpages that provide a link to the Legal Notice page. | |
Retrosynthesis Analysis
AI-Powered Synthesis Planning: Our tool employs the Template_relevance Pistachio, Template_relevance Bkms_metabolic, Template_relevance Pistachio_ringbreaker, Template_relevance Reaxys, Template_relevance Reaxys_biocatalysis model, leveraging a vast database of chemical reactions to predict feasible synthetic routes.
One-Step Synthesis Focus: Specifically designed for one-step synthesis, it provides concise and direct routes for your target compounds, streamlining the synthesis process.
Accurate Predictions: Utilizing the extensive PISTACHIO, BKMS_METABOLIC, PISTACHIO_RINGBREAKER, REAXYS, REAXYS_BIOCATALYSIS database, our tool offers high-accuracy predictions, reflecting the latest in chemical research and data.
Strategy Settings
Precursor scoring | Relevance Heuristic |
---|---|
Min. plausibility | 0.01 |
Model | Template_relevance |
Template Set | Pistachio/Bkms_metabolic/Pistachio_ringbreaker/Reaxys/Reaxys_biocatalysis |
Top-N result to add to graph | 6 |
Feasible Synthetic Routes
Disclaimer and Information on In-Vitro Research Products
Please be aware that all articles and product information presented on BenchChem are intended solely for informational purposes. The products available for purchase on BenchChem are specifically designed for in-vitro studies, which are conducted outside of living organisms. In-vitro studies, derived from the Latin term "in glass," involve experiments performed in controlled laboratory settings using cells or tissues. It is important to note that these products are not categorized as medicines or drugs, and they have not received approval from the FDA for the prevention, treatment, or cure of any medical condition, ailment, or disease. We must emphasize that any form of bodily introduction of these products into humans or animals is strictly prohibited by law. It is essential to adhere to these guidelines to ensure compliance with legal and ethical standards in research and experimentation.