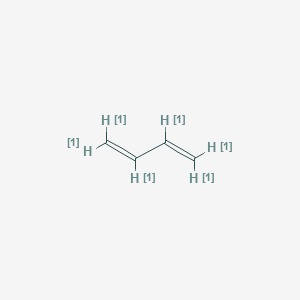
1,3-Butadiene, hexadeutero-, trans-
Overview
Description
1,3-Butadiene, hexadeutero-, trans- is a deuterated form of 1,3-butadiene, where all hydrogen atoms are replaced with deuterium. Its molecular formula is C4D6, and it has a molecular weight of 60.1274 g/mol . This compound is particularly useful in scientific research due to its unique isotopic properties, which allow for detailed studies of reaction mechanisms and molecular interactions.
Preparation Methods
Synthetic Routes and Reaction Conditions
1,3-Butadiene, hexadeutero-, trans- can be synthesized through the deuteration of 1,3-butadiene. This process typically involves the use of deuterium gas (D2) in the presence of a catalyst. The reaction conditions often include elevated temperatures and pressures to facilitate the exchange of hydrogen atoms with deuterium atoms.
Industrial Production Methods
Industrial production of 1,3-butadiene, hexadeutero-, trans- follows similar principles but on a larger scale. The process involves the catalytic deuteration of 1,3-butadiene using deuterium gas. The catalysts used in industrial settings are often more robust and efficient to ensure high yields and purity of the final product.
Chemical Reactions Analysis
Types of Reactions
1,3-Butadiene, hexadeutero-, trans- undergoes various chemical reactions, including:
Oxidation: This reaction involves the addition of oxygen to the compound, leading to the formation of deuterated oxidation products.
Reduction: Reduction reactions typically involve the addition of hydrogen or deuterium, resulting in the formation of deuterated alkanes.
Substitution: In substitution reactions, one or more deuterium atoms can be replaced by other atoms or groups, leading to the formation of various deuterated derivatives.
Common Reagents and Conditions
Oxidation: Common oxidizing agents include potassium permanganate (KMnO4) and ozone (O3). These reactions are usually carried out under controlled conditions to prevent over-oxidation.
Reduction: Catalytic hydrogenation using palladium (Pd) or platinum (Pt) catalysts is a common method for reducing 1,3-butadiene, hexadeutero-, trans-.
Substitution: Halogenation reactions using halogens (e.g., chlorine, bromine) or halogenating agents (e.g., N-bromosuccinimide) are typical substitution reactions.
Major Products Formed
Oxidation: Deuterated aldehydes, ketones, and carboxylic acids.
Reduction: Deuterated butanes and butenes.
Substitution: Deuterated halides and other substituted derivatives.
Scientific Research Applications
1,3-Butadiene, hexadeutero-, trans- has numerous applications in scientific research:
Chemistry: It is used to study reaction mechanisms and kinetics, particularly in the context of isotopic effects.
Biology: Deuterated compounds are used in metabolic studies to trace biochemical pathways.
Medicine: It is used in the development of deuterated drugs, which often exhibit improved pharmacokinetic properties.
Industry: It is used in the production of deuterated polymers and materials with unique properties.
Mechanism of Action
The mechanism of action of 1,3-butadiene, hexadeutero-, trans- involves its interaction with various molecular targets and pathways. The presence of deuterium atoms affects the bond strengths and reaction kinetics, leading to unique isotopic effects. These effects are particularly useful in studying detailed reaction mechanisms and molecular interactions.
Comparison with Similar Compounds
Similar Compounds
1,3-Butadiene: The non-deuterated form of the compound.
1,3-Butadiene, hexadeutero-, cis-: The cis-isomer of the deuterated compound.
1,3-Butadiene, tetradeutero-: A partially deuterated form with four deuterium atoms.
Uniqueness
1,3-Butadiene, hexadeutero-, trans- is unique due to its complete deuteration and trans-configuration. This makes it particularly valuable in studies requiring precise isotopic labeling and in understanding the effects of isotopic substitution on chemical and physical properties.
Properties
CAS No. |
1441-56-1 |
---|---|
Molecular Formula |
C4H6 |
Molecular Weight |
60.13 g/mol |
IUPAC Name |
1,1,2,3,4,4-hexadeuteriobuta-1,3-diene |
InChI |
InChI=1S/C4H6/c1-3-4-2/h3-4H,1-2H2/i1D2,2D2,3D,4D |
InChI Key |
KAKZBPTYRLMSJV-UFSLNRCZSA-N |
SMILES |
C=CC=C |
Isomeric SMILES |
[2H]C(=C([2H])C(=C([2H])[2H])[2H])[2H] |
Canonical SMILES |
C=CC=C |
Pictograms |
Flammable; Compressed Gas; Health Hazard |
Related CAS |
29989-19-3 |
Origin of Product |
United States |
Synthesis routes and methods I
Procedure details
Synthesis routes and methods II
Procedure details
Synthesis routes and methods III
Procedure details
Synthesis routes and methods IV
Procedure details
Synthesis routes and methods V
Procedure details
Disclaimer and Information on In-Vitro Research Products
Please be aware that all articles and product information presented on BenchChem are intended solely for informational purposes. The products available for purchase on BenchChem are specifically designed for in-vitro studies, which are conducted outside of living organisms. In-vitro studies, derived from the Latin term "in glass," involve experiments performed in controlled laboratory settings using cells or tissues. It is important to note that these products are not categorized as medicines or drugs, and they have not received approval from the FDA for the prevention, treatment, or cure of any medical condition, ailment, or disease. We must emphasize that any form of bodily introduction of these products into humans or animals is strictly prohibited by law. It is essential to adhere to these guidelines to ensure compliance with legal and ethical standards in research and experimentation.