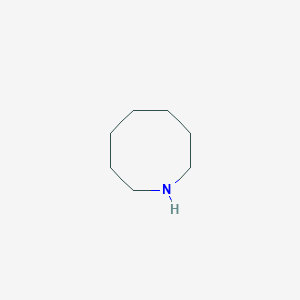
Azocane
Overview
Description
Azocane (1-azacyclooctane) is an eight-membered saturated N-heterocyclic compound. Synthetic this compound derivatives are explored for diverse applications, including acetylcholinesterase (AChE) inhibition, neuroprotection, and catalysis. Its eight-membered ring confers unique conformational flexibility and steric bulk, differentiating it from smaller N-heterocycles like piperidine or pyrrolidine .
Preparation Methods
Azocane can be synthesized through several methods:
Cyclization of 1,7-diaminoheptane: This method involves the intramolecular cyclization of 1,7-diaminoheptane under acidic conditions to form heptamethyleneimine.
Reduction of this compound: This compound can be reduced using hydrogen in the presence of a metal catalyst to yield heptamethyleneimine.
Industrial production: Industrially, heptamethyleneimine is produced by the catalytic hydrogenation of this compound, which is derived from cycloheptanone through a series of chemical transformations.
Chemical Reactions Analysis
Azocane undergoes various chemical reactions, including:
Oxidation: It can be oxidized to form heptamethyleneimine N-oxide using oxidizing agents such as hydrogen peroxide or peracids.
Reduction: Reduction of heptamethyleneimine can yield secondary amines or primary amines depending on the conditions and reagents used.
Common reagents and conditions: Typical reagents include hydrogen, metal catalysts, oxidizing agents, and nucleophiles.
Scientific Research Applications
Chemical Synthesis and Catalysis
Azocane derivatives have been utilized in several synthetic methodologies, particularly in the context of rhodium-catalyzed reactions. These reactions allow for the modular access to substituted azocanes through cycloaddition and fragmentation processes. For example, a study demonstrated a rhodium-catalyzed cycloaddition that efficiently produces azocanes from alkenes and other substrates, highlighting the compound's utility in organic synthesis .
Table 1: Rhodium-Catalyzed Reactions Involving this compound
This compound and its derivatives have shown promise in medicinal chemistry, particularly in antiviral applications. Notably, this compound derivatives have been investigated for their efficacy against hepatitis B virus (HBV). A patent outlines compounds derived from this compound that exhibit antiviral activity, suggesting their potential as therapeutic agents for HBV infections .
Case Study: Antiviral Activity of this compound Derivatives
- Objective : Evaluate the effectiveness of this compound derivatives against HBV.
- Method : In vitro testing of various this compound compounds.
- Findings : Certain derivatives exhibited significant antiviral activity, indicating their potential for drug development.
Structural Properties and Encapsulation Studies
Research has also focused on the structural characteristics of this compound, particularly its behavior in constrained environments. Studies utilizing molecular encapsulation techniques have explored how this compound interacts within non-polar solvents, providing insights into its stability and reactivity under different conditions .
Table 2: Structural Characteristics of this compound
Property | Description |
---|---|
Ring Structure | Bicyclic (8-membered ring) |
Strain Characteristics | Highly strained with unique geometries |
Solvent Interaction | Stable in non-polar environments |
Isolation and Natural Sources
This compound alkaloids have been isolated from various natural sources, revealing their bioactive properties. A review highlighted the structural diversity and biological activity of these alkaloids, suggesting that they could serve as lead compounds for further pharmacological development .
Table 3: Natural Sources of this compound Alkaloids
Source | Type of Alkaloid | Bioactivity |
---|---|---|
Plant Species A | Azocine derivatives | Antimicrobial |
Plant Species B | This compound alkaloids | Antiviral |
Mechanism of Action
The mechanism of action of heptamethyleneimine and its derivatives involves interactions with various molecular targets:
Comparison with Similar Compounds
Structural Comparison with Similar N-Heterocyclic Compounds
Azocane belongs to the family of saturated N-heterocycles, which vary in ring size and functional properties:
Key Observations :
- Synthesis: this compound derivatives are synthetically demanding compared to smaller rings. For example, the yield for this compound 29 (via enolate 1,4-addition) was modest (unpublished, cited in ), yet superior to prior methods .
- Conformational Flexibility : this compound mimics extended peptide conformers (e.g., C(+) conformer of ox-[Cys-Cys]), making it valuable in peptidomimetics .
Functional Comparison in Therapeutic Contexts
Acetylcholinesterase (AChE) Inhibition
This compound-containing xanthine derivatives demonstrate superior AChE inhibition:
The bulkier this compound moiety enhances steric complementarity with AChE’s active site .
Neuroprotection and Cognition Enhancement
This compound analogs of piracetam-like nootropics show retained or improved potency:
- Expanding piperidine (6-membered) to this compound (8-membered) in MN19 derivatives preserved antiamnesic activity in rodent models .
- This compound’s larger ring may improve binding to undefined neural targets, though mechanistic clarity remains lacking .
Toxicity vs. Therapeutic Utility
While natural this compound-containing alkaloids (e.g., otonecine) are hepatotoxic and genotoxic, synthetic derivatives mitigate risks through targeted modifications (e.g., non-peptidic XIAP antagonists) .
Role in Catalysis and Material Science
This compound serves as a ligand in transition-metal catalysts:
Biological Activity
Azocane, a saturated eight-membered nitrogen-containing heterocycle, has garnered attention due to its unique structural properties and biological activities. This article explores the synthesis, biological significance, and various research findings related to this compound, supported by case studies and data tables.
1. Structural Characteristics of this compound
This compound is characterized by its cyclic structure that includes a nitrogen atom in an eight-membered ring. This unique configuration allows for diverse chemical reactivity and interactions with biological systems. The compound can exist in multiple stereoisomeric forms, which may exhibit different biological activities.
2. Synthesis of Azocanes
Recent advancements in synthetic methodologies have enabled the efficient production of azocanes. For instance, azocanes can be synthesized from piperidine derivatives through ring-expansion reactions involving azetidinium intermediates. These reactions often utilize nucleophiles like primary and secondary amines, yielding azocanes with high diastereoselectivity .
Table 1: Synthetic Methods for this compound Production
Methodology | Yield (%) | Notes |
---|---|---|
Ring-expansion from piperidines | 60-74 | High diastereoselectivity achieved |
Rhodium-catalyzed cycloaddition | 34-53 | Effective under specific conditions |
Enantioselective synthesis | 63-97 | Achieved through enzymatic resolution |
3. Biological Activity
The biological activity of this compound derivatives has been explored in various studies, particularly focusing on their potential as therapeutic agents. Research indicates that this compound-containing compounds exhibit a range of pharmacological effects, including anti-cancer properties and interactions with specific biological targets.
Case Study: Anticancer Activity
A study investigating the biological activity of this compound alkaloids demonstrated significant cytotoxic effects against cancer cell lines. The mechanisms involved include the inhibition of cell proliferation and induction of apoptosis. The presence of specific substituents on the this compound ring was found to enhance these effects, highlighting the importance of structural modifications .
Table 2: Biological Activities of this compound Derivatives
Compound | Activity Type | IC50 (µM) | Reference |
---|---|---|---|
This compound A | Cytotoxicity | 15 | |
This compound B | Antimicrobial | 25 | |
This compound C | Anti-inflammatory | 30 |
4. Mechanistic Insights
The biological effects of azocanes are often linked to their ability to interact with specific proteins or enzymes within biological pathways. For example, certain this compound derivatives have been shown to act as inhibitors of key enzymes involved in cancer progression or inflammation .
5. Future Directions in Research
The ongoing exploration of azocanes in medicinal chemistry is promising. Future research may focus on:
- Optimization of Synthesis : Developing more efficient synthetic routes to produce diverse this compound derivatives.
- Mechanistic Studies : Elucidating the precise mechanisms by which azocanes exert their biological effects.
- Clinical Applications : Investigating the potential for azocanes in clinical settings, particularly in oncology and infectious diseases.
Q & A
Basic Research Questions
Q. What are the established synthetic methodologies for azocane and its derivatives, and how can researchers optimize reaction conditions for improved yields?
this compound, an 8-membered saturated nitrogen-containing heterocycle, is typically synthesized via cyclization of linear precursors or functionalization of smaller heterocycles. For example, in sulfonamide synthesis, this compound derivatives are obtained by reacting this compound with thiols under palladium catalysis, yielding products with moderate to good efficiency (Table 3, ). Optimization strategies include:
- Systematic variation of catalysts (e.g., Pd(OAc)₂ vs. PdCl₂).
- Temperature gradients (e.g., 80–120°C) to assess reaction kinetics.
- Solvent polarity screening (e.g., DMF vs. THF) to stabilize intermediates. Researchers should cross-reference synthetic protocols from peer-reviewed journals and validate reproducibility through triplicate trials .
Q. Which spectroscopic techniques are most effective for characterizing this compound derivatives, and how should data interpretation be approached?
Key techniques include:
- ¹H/¹³C NMR : To confirm ring structure and substituent positions. This compound’s conformational flexibility may lead to split signals, requiring high-resolution NMR (400+ MHz) .
- Mass Spectrometry (HRMS) : To verify molecular ion peaks and fragmentation patterns.
- X-ray Crystallography : For unambiguous structural determination, particularly for novel derivatives. Data interpretation should align with computational predictions (e.g., DFT-calculated chemical shifts) to resolve ambiguities .
Advanced Research Questions
Q. How can computational chemistry techniques predict the reactivity and stability of this compound-containing compounds in drug design?
Computational methods such as:
- Density Functional Theory (DFT) : To calculate ring strain, electron density maps, and reaction transition states.
- Molecular Dynamics (MD) Simulations : To assess solvation effects and protein-ligand binding kinetics. For instance, this compound’s larger ring size compared to piperidine may reduce steric hindrance in enzyme active sites, enhancing binding affinity. Researchers should validate computational models with experimental data (e.g., IC₅₀ values) and use software like Gaussian or Schrödinger Suite .
Q. What strategies resolve discrepancies in reported reaction yields or mechanistic pathways involving this compound?
Contradictory data may arise from:
- Impurity profiles : Use HPLC or GC-MS to quantify byproducts.
- Reaction conditions : Replicate studies under strictly controlled parameters (e.g., inert atmosphere, moisture-free solvents).
- Mechanistic probes : Isotopic labeling (e.g., ¹⁵N-azocane) to track reaction pathways. Cross-disciplinary collaboration with statisticians can strengthen data analysis rigor (e.g., ANOVA for yield comparisons) .
Q. How does this compound’s ring size influence its chemical behavior compared to smaller heterocycles like piperidine?
this compound’s 8-membered ring exhibits:
- Reduced ring strain : Enables unique conformations (e.g., boat vs. chair).
- Enhanced flexibility : May improve binding entropy in host-guest systems. Comparative studies should employ:
- Kinetic assays : To measure nucleophilic substitution rates.
- Thermodynamic analyses : DSC/TGA for stability profiling. Patent literature highlights this compound’s preferential use over piperidine in certain catalytic applications (e.g., EP 4 374 877 A2) .
Q. Methodological Guidance
Q. What experimental design principles are critical for studying this compound’s catalytic applications?
Key considerations include:
- Control experiments : Baseline reactions without catalysts or this compound derivatives.
- Multivariate analysis : DOE (Design of Experiments) to isolate variable impacts.
- Literature benchmarking : Compare results against analogous systems (e.g., azepane or morpholine derivatives). Transparent reporting of raw data and reproducibility checks (e.g., Open Science Framework repositories) are essential .
Q. How can researchers ensure ethical and rigorous literature reviews when investigating this compound’s applications?
Apply the FINER framework :
- Feasible : Prioritize accessible journals (e.g., Reviews in Analytical Chemistry) over paywalled sources.
- Novel : Identify gaps (e.g., limited studies on this compound’s photostability).
- Ethical : Cite conflicting findings transparently; avoid selective reporting. Librarians and methodologists should be consulted to mitigate bias in source selection .
Properties
IUPAC Name |
azocane | |
---|---|---|
Source | PubChem | |
URL | https://pubchem.ncbi.nlm.nih.gov | |
Description | Data deposited in or computed by PubChem | |
InChI |
InChI=1S/C7H15N/c1-2-4-6-8-7-5-3-1/h8H,1-7H2 | |
Source | PubChem | |
URL | https://pubchem.ncbi.nlm.nih.gov | |
Description | Data deposited in or computed by PubChem | |
InChI Key |
QXNDZONIWRINJR-UHFFFAOYSA-N | |
Source | PubChem | |
URL | https://pubchem.ncbi.nlm.nih.gov | |
Description | Data deposited in or computed by PubChem | |
Canonical SMILES |
C1CCCNCCC1 | |
Source | PubChem | |
URL | https://pubchem.ncbi.nlm.nih.gov | |
Description | Data deposited in or computed by PubChem | |
Molecular Formula |
C7H15N | |
Source | PubChem | |
URL | https://pubchem.ncbi.nlm.nih.gov | |
Description | Data deposited in or computed by PubChem | |
DSSTOX Substance ID |
DTXSID2020680 | |
Record name | Heptamethyleneimine | |
Source | EPA DSSTox | |
URL | https://comptox.epa.gov/dashboard/DTXSID2020680 | |
Description | DSSTox provides a high quality public chemistry resource for supporting improved predictive toxicology. | |
Molecular Weight |
113.20 g/mol | |
Source | PubChem | |
URL | https://pubchem.ncbi.nlm.nih.gov | |
Description | Data deposited in or computed by PubChem | |
CAS No. |
1121-92-2 | |
Record name | Azocan | |
Source | CAS Common Chemistry | |
URL | https://commonchemistry.cas.org/detail?cas_rn=1121-92-2 | |
Description | CAS Common Chemistry is an open community resource for accessing chemical information. Nearly 500,000 chemical substances from CAS REGISTRY cover areas of community interest, including common and frequently regulated chemicals, and those relevant to high school and undergraduate chemistry classes. This chemical information, curated by our expert scientists, is provided in alignment with our mission as a division of the American Chemical Society. | |
Explanation | The data from CAS Common Chemistry is provided under a CC-BY-NC 4.0 license, unless otherwise stated. | |
Record name | Heptamethyleneimine | |
Source | ChemIDplus | |
URL | https://pubchem.ncbi.nlm.nih.gov/substance/?source=chemidplus&sourceid=0001121922 | |
Description | ChemIDplus is a free, web search system that provides access to the structure and nomenclature authority files used for the identification of chemical substances cited in National Library of Medicine (NLM) databases, including the TOXNET system. | |
Record name | Azocine, octahydro- | |
Source | EPA Chemicals under the TSCA | |
URL | https://www.epa.gov/chemicals-under-tsca | |
Description | EPA Chemicals under the Toxic Substances Control Act (TSCA) collection contains information on chemicals and their regulations under TSCA, including non-confidential content from the TSCA Chemical Substance Inventory and Chemical Data Reporting. | |
Record name | Heptamethyleneimine | |
Source | EPA DSSTox | |
URL | https://comptox.epa.gov/dashboard/DTXSID2020680 | |
Description | DSSTox provides a high quality public chemistry resource for supporting improved predictive toxicology. | |
Record name | Perhydroazocine | |
Source | European Chemicals Agency (ECHA) | |
URL | https://echa.europa.eu/substance-information/-/substanceinfo/100.013.039 | |
Description | The European Chemicals Agency (ECHA) is an agency of the European Union which is the driving force among regulatory authorities in implementing the EU's groundbreaking chemicals legislation for the benefit of human health and the environment as well as for innovation and competitiveness. | |
Explanation | Use of the information, documents and data from the ECHA website is subject to the terms and conditions of this Legal Notice, and subject to other binding limitations provided for under applicable law, the information, documents and data made available on the ECHA website may be reproduced, distributed and/or used, totally or in part, for non-commercial purposes provided that ECHA is acknowledged as the source: "Source: European Chemicals Agency, http://echa.europa.eu/". Such acknowledgement must be included in each copy of the material. ECHA permits and encourages organisations and individuals to create links to the ECHA website under the following cumulative conditions: Links can only be made to webpages that provide a link to the Legal Notice page. | |
Retrosynthesis Analysis
AI-Powered Synthesis Planning: Our tool employs the Template_relevance Pistachio, Template_relevance Bkms_metabolic, Template_relevance Pistachio_ringbreaker, Template_relevance Reaxys, Template_relevance Reaxys_biocatalysis model, leveraging a vast database of chemical reactions to predict feasible synthetic routes.
One-Step Synthesis Focus: Specifically designed for one-step synthesis, it provides concise and direct routes for your target compounds, streamlining the synthesis process.
Accurate Predictions: Utilizing the extensive PISTACHIO, BKMS_METABOLIC, PISTACHIO_RINGBREAKER, REAXYS, REAXYS_BIOCATALYSIS database, our tool offers high-accuracy predictions, reflecting the latest in chemical research and data.
Strategy Settings
Precursor scoring | Relevance Heuristic |
---|---|
Min. plausibility | 0.01 |
Model | Template_relevance |
Template Set | Pistachio/Bkms_metabolic/Pistachio_ringbreaker/Reaxys/Reaxys_biocatalysis |
Top-N result to add to graph | 6 |
Feasible Synthetic Routes
Disclaimer and Information on In-Vitro Research Products
Please be aware that all articles and product information presented on BenchChem are intended solely for informational purposes. The products available for purchase on BenchChem are specifically designed for in-vitro studies, which are conducted outside of living organisms. In-vitro studies, derived from the Latin term "in glass," involve experiments performed in controlled laboratory settings using cells or tissues. It is important to note that these products are not categorized as medicines or drugs, and they have not received approval from the FDA for the prevention, treatment, or cure of any medical condition, ailment, or disease. We must emphasize that any form of bodily introduction of these products into humans or animals is strictly prohibited by law. It is essential to adhere to these guidelines to ensure compliance with legal and ethical standards in research and experimentation.