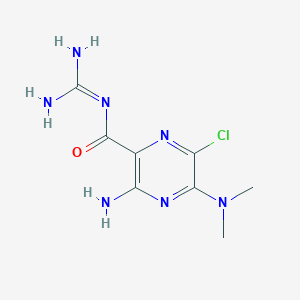
5-Dimethylamiloride
Overview
Description
5-Dimethylamiloride (also known as 5-DMA, dimethylamiloride, MK 685, MK-685) is a chemical compound with the molecular formula C8H12ClN7O . It has a molecular weight of 257.68 g/mol . The IUPAC name for this compound is 3-amino-6-chloro-N-(diaminomethylidene)-5-(dimethylamino)pyrazine-2-carboxamide .
Molecular Structure Analysis
The molecular structure of 5-Dimethylamiloride can be represented by the following canonical SMILES: CN©C1=NC(=C(N=C1Cl)C(=O)N=C(N)N)N . This representation provides a text notation for the compound’s structure, which includes information about the arrangement of atoms and the bonds between them .
Scientific Research Applications
Cellular Biology: Na+/H+ Antiport Inhibition
5-Dimethylamiloride (DMA) is known for its role as a selective inhibitor of the Na+/H+ antiport . This antiporter plays a crucial role in regulating intracellular pH and cell volume. By inhibiting this antiporter, DMA can be used to study cellular processes related to pH regulation, such as cell proliferation, apoptosis, and migration.
Pharmacology: Pain Management
In pharmacological research, DMA has been investigated for its potential to reduce inflammatory pain . Its ability to modulate ion transport across cellular membranes makes it a candidate for developing new analgesic drugs.
Molecular Biology: Enzyme Activity Studies
In molecular biology, DMA is utilized to study enzyme activities that are sensitive to intracellular pH changes. It serves as a tool to understand the enzymatic mechanisms that are pH-dependent .
Mechanism of Action
Target of Action
5-Dimethylamiloride, a derivative of Amiloride , primarily targets the Epithelial Sodium Channels (ENaC) . These channels play a crucial role in sodium reabsorption in the distal convoluted tubules and collecting ducts in the kidneys . By inhibiting these channels, 5-Dimethylamiloride affects the balance of sodium and potassium in the body .
Mode of Action
5-Dimethylamiloride works by inhibiting sodium reabsorption in the distal convoluted tubules and collecting ducts in the kidneys by binding to the amiloride-sensitive sodium channels . This promotes the loss of sodium and water from the body, but without depleting potassium . It also blocks the Na+/H+ exchangers such as sodium–hydrogen antiporter 1 (NHE-1) .
Biochemical Pathways
The inhibition of sodium reabsorption by 5-Dimethylamiloride creates a negative potential in the luminal membranes of principal cells, located in the distal convoluted tubule and collecting duct . This negative potential reduces the secretion of potassium and hydrogen ions . It also abolishes more than 80% of the action of angiotensin II on the secretion of hydrogen ions in the proximal tubule .
Pharmacokinetics
Amiloride, from which 5-Dimethylamiloride is derived, is readily absorbed with a bioavailability of 15–25% . It reaches its peak diuretic effect at 6–10 hours . It is 40% protein-bound and has a plasma half-life of 6–9 hours .
Result of Action
The primary result of 5-Dimethylamiloride’s action is an increase in sodium and water excretion from the body, while reducing potassium excretion . This can help manage conditions like hypertension and congestive heart failure . It also reduces the sensitivity of afferent fibres to electrical stimulation, requiring greater stimulation voltages to induce action potentials from their peripheral terminals within the trachea/bronchus .
Action Environment
The action of 5-Dimethylamiloride can be influenced by various environmental factors. For instance, its action is prolonged in renal impairment and is contraindicated if eGFR is <10ml/min/1.73 m2 . The risk of hyperkalemia increases in the presence of renal impairment, diabetes, or co-administration with other potassium-sparing diuretics, ACE inhibitors, and angiotensin receptor blockers .
Future Directions
properties
IUPAC Name |
3-amino-6-chloro-N-(diaminomethylidene)-5-(dimethylamino)pyrazine-2-carboxamide | |
---|---|---|
Source | PubChem | |
URL | https://pubchem.ncbi.nlm.nih.gov | |
Description | Data deposited in or computed by PubChem | |
InChI |
InChI=1S/C8H12ClN7O/c1-16(2)6-4(9)13-3(5(10)14-6)7(17)15-8(11)12/h1-2H3,(H2,10,14)(H4,11,12,15,17) | |
Source | PubChem | |
URL | https://pubchem.ncbi.nlm.nih.gov | |
Description | Data deposited in or computed by PubChem | |
InChI Key |
RXMUPNVSYKGKMY-UHFFFAOYSA-N | |
Source | PubChem | |
URL | https://pubchem.ncbi.nlm.nih.gov | |
Description | Data deposited in or computed by PubChem | |
Canonical SMILES |
CN(C)C1=NC(=C(N=C1Cl)C(=O)N=C(N)N)N | |
Source | PubChem | |
URL | https://pubchem.ncbi.nlm.nih.gov | |
Description | Data deposited in or computed by PubChem | |
Molecular Formula |
C8H12ClN7O | |
Source | PubChem | |
URL | https://pubchem.ncbi.nlm.nih.gov | |
Description | Data deposited in or computed by PubChem | |
DSSTOX Substance ID |
DTXSID70923797 | |
Record name | 5-Dimethylamiloride | |
Source | EPA DSSTox | |
URL | https://comptox.epa.gov/dashboard/DTXSID70923797 | |
Description | DSSTox provides a high quality public chemistry resource for supporting improved predictive toxicology. | |
Molecular Weight |
257.68 g/mol | |
Source | PubChem | |
URL | https://pubchem.ncbi.nlm.nih.gov | |
Description | Data deposited in or computed by PubChem | |
Product Name |
5-Dimethylamiloride | |
CAS RN |
1214-79-5 | |
Record name | 5-Dimethylamiloride | |
Source | ChemIDplus | |
URL | https://pubchem.ncbi.nlm.nih.gov/substance/?source=chemidplus&sourceid=0001214795 | |
Description | ChemIDplus is a free, web search system that provides access to the structure and nomenclature authority files used for the identification of chemical substances cited in National Library of Medicine (NLM) databases, including the TOXNET system. | |
Record name | 5-Dimethylamiloride | |
Source | EPA DSSTox | |
URL | https://comptox.epa.gov/dashboard/DTXSID70923797 | |
Description | DSSTox provides a high quality public chemistry resource for supporting improved predictive toxicology. | |
Record name | 1214-79-5 | |
Source | European Chemicals Agency (ECHA) | |
URL | https://echa.europa.eu/information-on-chemicals | |
Description | The European Chemicals Agency (ECHA) is an agency of the European Union which is the driving force among regulatory authorities in implementing the EU's groundbreaking chemicals legislation for the benefit of human health and the environment as well as for innovation and competitiveness. | |
Explanation | Use of the information, documents and data from the ECHA website is subject to the terms and conditions of this Legal Notice, and subject to other binding limitations provided for under applicable law, the information, documents and data made available on the ECHA website may be reproduced, distributed and/or used, totally or in part, for non-commercial purposes provided that ECHA is acknowledged as the source: "Source: European Chemicals Agency, http://echa.europa.eu/". Such acknowledgement must be included in each copy of the material. ECHA permits and encourages organisations and individuals to create links to the ECHA website under the following cumulative conditions: Links can only be made to webpages that provide a link to the Legal Notice page. | |
Record name | DIMETHYLAMILORIDE | |
Source | FDA Global Substance Registration System (GSRS) | |
URL | https://gsrs.ncats.nih.gov/ginas/app/beta/substances/3GC547293P | |
Description | The FDA Global Substance Registration System (GSRS) enables the efficient and accurate exchange of information on what substances are in regulated products. Instead of relying on names, which vary across regulatory domains, countries, and regions, the GSRS knowledge base makes it possible for substances to be defined by standardized, scientific descriptions. | |
Explanation | Unless otherwise noted, the contents of the FDA website (www.fda.gov), both text and graphics, are not copyrighted. They are in the public domain and may be republished, reprinted and otherwise used freely by anyone without the need to obtain permission from FDA. Credit to the U.S. Food and Drug Administration as the source is appreciated but not required. | |
Retrosynthesis Analysis
AI-Powered Synthesis Planning: Our tool employs the Template_relevance Pistachio, Template_relevance Bkms_metabolic, Template_relevance Pistachio_ringbreaker, Template_relevance Reaxys, Template_relevance Reaxys_biocatalysis model, leveraging a vast database of chemical reactions to predict feasible synthetic routes.
One-Step Synthesis Focus: Specifically designed for one-step synthesis, it provides concise and direct routes for your target compounds, streamlining the synthesis process.
Accurate Predictions: Utilizing the extensive PISTACHIO, BKMS_METABOLIC, PISTACHIO_RINGBREAKER, REAXYS, REAXYS_BIOCATALYSIS database, our tool offers high-accuracy predictions, reflecting the latest in chemical research and data.
Strategy Settings
Precursor scoring | Relevance Heuristic |
---|---|
Min. plausibility | 0.01 |
Model | Template_relevance |
Template Set | Pistachio/Bkms_metabolic/Pistachio_ringbreaker/Reaxys/Reaxys_biocatalysis |
Top-N result to add to graph | 6 |
Feasible Synthetic Routes
Disclaimer and Information on In-Vitro Research Products
Please be aware that all articles and product information presented on BenchChem are intended solely for informational purposes. The products available for purchase on BenchChem are specifically designed for in-vitro studies, which are conducted outside of living organisms. In-vitro studies, derived from the Latin term "in glass," involve experiments performed in controlled laboratory settings using cells or tissues. It is important to note that these products are not categorized as medicines or drugs, and they have not received approval from the FDA for the prevention, treatment, or cure of any medical condition, ailment, or disease. We must emphasize that any form of bodily introduction of these products into humans or animals is strictly prohibited by law. It is essential to adhere to these guidelines to ensure compliance with legal and ethical standards in research and experimentation.