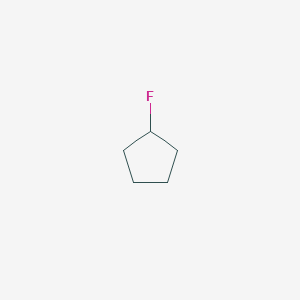
Fluorocyclopentane
Overview
Description
Fluorocyclopentane (C₅H₉F) is a fluorinated derivative of cyclopentane, where one hydrogen atom is replaced by fluorine. Its molecular weight is 88.12 g/mol, with a density of 0.9 g/cm³ and a boiling point of 68.8°C at 760 mmHg . The compound exhibits a unique conformational landscape due to the electronegative fluorine atom, which stabilizes specific conformers. Early studies proposed a single stable envelope-equatorial (1E) conformer, but advanced computational methods revealed two dominant minima: the 5T4/5E and 2T3/2E conformations, with the pseudo-axial fluorine conformation (E1) being 1.33–1.59 kcal/mol more stable than the pseudo-equatorial (1E) form .
This compound is utilized in industrial applications, including electronics cleaning and azeotropic mixtures, as highlighted by its role in Sinochem Group’s award-winning project for sustainable manufacturing .
Preparation Methods
Synthetic Routes and Reaction Conditions: Fluorocyclopentane can be synthesized through several methods. One common approach involves the fluorination of cyclopentane using fluorinating agents such as hydrogen fluoride or elemental fluorine. The reaction typically occurs under controlled conditions to ensure selective fluorination and to avoid over-fluorination or decomposition of the product.
Industrial Production Methods: In industrial settings, this compound is produced using large-scale fluorination processes. These processes often involve the use of specialized reactors and catalysts to achieve high yields and purity. The reaction conditions, such as temperature, pressure, and the concentration of fluorinating agents, are carefully optimized to maximize efficiency and minimize by-products.
Chemical Reactions Analysis
Types of Reactions: Fluorocyclopentane undergoes various chemical reactions, including:
Substitution Reactions: The fluorine atom in this compound can be replaced by other substituents through nucleophilic substitution reactions. Common reagents for these reactions include alkali metals and organometallic compounds.
Oxidation Reactions: this compound can be oxidized to form fluorinated cyclopentanones or cyclopentanol derivatives. Oxidizing agents such as potassium permanganate or chromium trioxide are typically used.
Reduction Reactions: The compound can be reduced to form cyclopentane or other partially fluorinated cyclopentane derivatives. Reducing agents like lithium aluminum hydride or sodium borohydride are commonly employed.
Common Reagents and Conditions:
Substitution: Alkali metals, organometallic compounds, solvents like tetrahydrofuran or dimethyl sulfoxide.
Oxidation: Potassium permanganate, chromium trioxide, solvents like acetone or dichloromethane.
Reduction: Lithium aluminum hydride, sodium borohydride, solvents like ether or tetrahydrofuran.
Major Products:
Substitution: Various substituted cyclopentane derivatives.
Oxidation: Fluorinated cyclopentanones, cyclopentanol derivatives.
Reduction: Cyclopentane, partially fluorinated cyclopentane derivatives.
Scientific Research Applications
Chemical Synthesis
Fluorocyclopentane is primarily utilized as a building block in the synthesis of more complex fluorinated organic compounds. Its structure allows researchers to explore reaction mechanisms and kinetics effectively. The introduction of fluorine can significantly alter the reactivity and stability of organic molecules, making this compound a valuable intermediate in synthetic pathways.
Key Uses in Chemical Synthesis
- Building Block : Serves as a precursor for synthesizing other fluorinated compounds.
- Mechanistic Studies : Used in investigations of reaction mechanisms and kinetics.
Biological Applications
In biology, this compound has been investigated for its potential as a biological imaging agent and as a probe in biochemical assays. The unique properties of the fluorine atom enhance the visibility of compounds in imaging techniques such as Positron Emission Tomography (PET).
Case Study: PET Imaging
A notable application involves the development of 4-fluorocyclopentane-1-carboxylic acid derivatives, which have shown promising results in imaging gliomas and prostate cancer. These derivatives demonstrated good brain uptake and higher tumor-to-brain tissue ratios compared to traditional imaging agents like -FDG, indicating their potential utility in clinical diagnostics .
Pharmaceutical Development
This compound is also explored for its role in drug development . The incorporation of fluorine into drug molecules can enhance metabolic stability and bioavailability, leading to more effective therapeutic agents.
Pharmaceutical Insights
- Fluorinated Pharmaceuticals : Compounds derived from this compound are being studied for their effectiveness against various diseases, including cancer.
- Stability Improvement : Fluorination can stabilize drug candidates against metabolic degradation.
Industrial Applications
In industrial settings, this compound is employed in the production of specialty chemicals, including fluorinated solvents and intermediates for agrochemicals. Its unique chemical properties make it suitable for various applications in materials science.
Industrial Uses
- Specialty Chemicals : Used as a solvent or intermediate in chemical manufacturing.
- Agrochemical Production : Contributes to the development of advanced agrochemical formulations.
Research Opportunities
- Further exploration of its biological interactions.
- Development of new derivatives for enhanced imaging capabilities.
- Investigation into its role in novel therapeutic agents.
Mechanism of Action
The mechanism of action of fluorocyclopentane involves its interaction with various molecular targets and pathways. The presence of the fluorine atom can significantly alter the compound’s reactivity and interaction with biological molecules. This compound can participate in hydrogen bonding, dipole-dipole interactions, and other non-covalent interactions, influencing its behavior in chemical and biological systems.
Comparison with Similar Compounds
Fluorocyclopentane vs. Fluorocyclohexane
Key Differences :
- Ring Size Effects : The smaller cyclopentane ring increases ring strain, leading to greater conformational flexibility compared to fluorocyclohexane’s rigid chair conformation.
- Fluorine Orientation : Pseudo-axial fluorine is stabilized in this compound due to hyperconjugation and reduced steric hindrance , whereas equatorial fluorine is favored in fluorocyclohexane .
This compound vs. Cyclopentane
Key Differences :
- Chemical Inertness : this compound’s C–F bond reduces susceptibility to radical reactions and oxidation compared to cyclopentane .
- Thermodynamic Stability : Fluorine substitution lowers vapor pressure (150 mmHg at 25°C ) relative to cyclopentane (317 mmHg).
This compound vs. 1-Fluoropentane
Key Differences :
- Ring vs. Chain Effects : this compound’s cyclic structure enhances thermal stability and dipole interactions, making it more suitable for clathrate formation .
- Synthetic Accessibility : 1-Fluoropentane is easier to synthesize via direct fluorination, whereas this compound requires ring-functionalization strategies .
This compound vs. Cyclopentene
Key Differences :
- Chemical Versatility : Cyclopentene’s double bond enables polymerization and Diels-Alder reactions, whereas this compound is primarily used for its inertness .
Biological Activity
Fluorocyclopentane and its derivatives, particularly fluorinated amino acids, have garnered attention in the field of molecular imaging and cancer diagnostics due to their unique biological properties. This article explores the biological activity of this compound, focusing on its applications in positron emission tomography (PET) imaging, particularly for prostate cancer, as well as its uptake mechanisms and biodistribution.
Overview of this compound
This compound is a cyclic compound that has been modified to include fluorine atoms, enhancing its utility in various biological applications. The most notable derivatives include 4-fluorocyclopentane-1-carboxylic acid (FACBC) and anti-2-fluorocyclopentane-1-carboxylic acid (FACPC), which have been studied extensively for their roles as radiotracers in PET imaging.
Mechanisms of Biological Activity
The biological activity of this compound derivatives primarily involves their uptake by cancer cells. Studies indicate that these compounds are transported into cells via specific amino acid transport systems, notably system L and system ASC.
Uptake Mechanisms
- System L Transport : This system is responsible for the transport of large neutral amino acids. This compound derivatives demonstrate significant uptake through this pathway, which is crucial for their effectiveness as radiotracers.
- System ASC Transport : This system facilitates the transport of small neutral amino acids and some other compounds. While the contribution is less pronounced than system L, it still plays a role in the cellular uptake of fluorinated compounds.
PET Imaging in Prostate Cancer
A significant body of research has focused on the use of this compound derivatives in imaging prostate carcinoma. For instance:
- Study on Anti-18F-FACBC : This study demonstrated that anti-18F-FACBC shows excellent uptake in prostate cancer cells, with a maximum standardized uptake value (SUVmax) of 4.1 ± 1.3 in malignant lesions at 5 minutes post-injection. Comparatively, anti-18F-FACBC had a lower blood pool activity, indicating better lesion visibility against background tissues .
Parameter | Anti-18F-FACBC | Anti-2-[18F]FACPC |
---|---|---|
SUVmax at 5 min | 4.1 ± 1.3 | 4.3 ± 1.1 |
Blood Pool SUVmean | 2.7 ± 0.5 | 1.3 ± 0.3 |
Bladder SUVmean at 20 min | 2.1 ± 1.2 | 42.2 ± 30.3 |
This table summarizes key findings from studies comparing different radiotracers based on their uptake characteristics.
Biodistribution Studies
Biodistribution studies have shown that while both anti-18F-FACBC and anti-2-[18F]FACPC exhibit high tumor uptake, they differ significantly in their accumulation patterns in non-target tissues:
Q & A
Basic Research Questions
Q. What experimental methods are most effective for characterizing the conformational landscape of fluorocyclopentane?
this compound exhibits multiple conformers due to fluorine substitution, such as envelope-equatorial (1E) and twist (5T4/5E) structures. Key methods include:
- Vibrational spectroscopy (e.g., IR/Raman) to identify stable conformers via vibrational mode analysis.
- Computational modeling (e.g., DFT, CNDO/2) to compare relative stabilities of conformers and transition states .
- X-ray crystallography for solid-state structural validation, though pseudorotation dynamics in solution complicate this approach.
Q. How does fluorination alter the thermodynamic stability of cyclopentane derivatives?
Fluorine’s electronegativity and axial preference in cycloalkanes introduce hyperconjugative and steric effects. For example:
- Pseudo-axial fluorine in E1 conformers stabilizes this compound by 1.33–1.59 kcal/mol compared to pseudo-equatorial arrangements due to reduced gauche interactions .
- Comparative studies with chlorocyclopentane highlight fluorine’s unique stereoelectronic effects on ring puckering.
Q. What synthetic routes are recommended for preparing this compound derivatives with high purity?
- Nucleophilic fluorination of cyclopentanol derivatives using DAST (diethylaminosulfur trifluoride).
- Gas-phase reactions (e.g., fluorine substitution via radical pathways) to minimize solvent interference.
- Characterization protocols : NMR (<sup>19</sup>F, <sup>1</sup>H) and GC-MS for purity validation, adhering to journal standards for new compound documentation .
Advanced Research Questions
Q. How can contradictions between historical and modern computational studies on this compound conformers be resolved?
Early vibrational studies proposed a single conformer (1E), while DFT calculations revealed multiple minima (e.g., E1 and 2T3/2E). Methodological considerations include:
- Basis set selection : Larger basis sets (e.g., cc-pVTZ) improve accuracy for weak hyperconjugative interactions.
- Dynamic vs. static analysis : Molecular dynamics simulations account for pseudorotation, unlike static calculations .
- Experimental validation : Low-temperature NMR to trap conformers transiently observed in computational models.
Q. What strategies mitigate challenges in quantifying this compound’s pseudorotation energy barriers?
Pseudorotation complicates energy barrier measurements. Solutions include:
- Variable-temperature studies : Plotting <sup>19</sup>F NMR chemical shifts against temperature to estimate ΔG‡.
- Transition state localization : Using QM/MM hybrid methods to map reaction coordinates between conformers.
- Comparative analysis : Contrasting barriers with non-fluorinated analogs to isolate fluorine’s role .
Q. How do solvent effects influence this compound’s conformational equilibrium in solution?
Solvent polarity and hydrogen-bonding capacity shift equilibria:
- Polar aprotic solvents (e.g., DMSO) stabilize pseudo-axial conformers via dipole-dipole interactions.
- MD simulations with explicit solvent models to quantify solvation shell impacts.
- Empirical validation via solvent-dependent <sup>19</sup>F NMR coupling constants .
Q. Methodological and Analytical Guidance
Q. What frameworks (e.g., PICOT, FINER) are suitable for designing this compound studies?
Adapt clinical research frameworks to chemistry:
- PICOT :
- Population : this compound derivatives.
- Intervention : Computational method (DFT vs. MP2).
- Comparison : Stability relative to chloro-/bromo- analogs.
- Outcome : Conformer distribution.
- Time : Simulation time vs. experimental validation .
Q. How should researchers address reproducibility challenges in this compound synthesis?
- Detailed protocols : Document reaction conditions (temperature, solvent purity, catalyst loading) in supplementary materials .
- Error analysis : Report uncertainties in spectroscopic data (e.g., ±0.01 ppm for <sup>19</sup>F NMR).
- Cross-lab validation : Collaborate to replicate results using independent instrumentation.
Q. Data Presentation and Peer Review
Q. What are best practices for presenting conformational analysis data in publications?
- Tables : Compare computed vs. experimental bond lengths, angles, and energies (see example below).
- Figures : Use 3D conformational maps with energy contours (e.g., Figure 3 in ).
- Supplementary data : Include raw computational output files and crystallographic CIFs .
Conformer | Energy (kcal/mol) | Method |
---|---|---|
E1 | 0.00 | DFT/B3LYP |
1E | 1.59 | CNDO/2 |
Q. How to critically evaluate conflicting data on this compound’s reactivity?
- Systematic reviews : Meta-analysis of kinetic data (e.g., fluorination rates under varying conditions).
- Sensitivity analysis : Test how computational parameters (e.g., solvent model) affect reaction pathway predictions.
- Peer feedback : Present preliminary findings at conferences to identify methodological gaps .
Properties
IUPAC Name |
fluorocyclopentane | |
---|---|---|
Source | PubChem | |
URL | https://pubchem.ncbi.nlm.nih.gov | |
Description | Data deposited in or computed by PubChem | |
InChI |
InChI=1S/C5H9F/c6-5-3-1-2-4-5/h5H,1-4H2 | |
Source | PubChem | |
URL | https://pubchem.ncbi.nlm.nih.gov | |
Description | Data deposited in or computed by PubChem | |
InChI Key |
YHYNFMGKZFOMAG-UHFFFAOYSA-N | |
Source | PubChem | |
URL | https://pubchem.ncbi.nlm.nih.gov | |
Description | Data deposited in or computed by PubChem | |
Canonical SMILES |
C1CCC(C1)F | |
Source | PubChem | |
URL | https://pubchem.ncbi.nlm.nih.gov | |
Description | Data deposited in or computed by PubChem | |
Molecular Formula |
C5H9F | |
Source | PubChem | |
URL | https://pubchem.ncbi.nlm.nih.gov | |
Description | Data deposited in or computed by PubChem | |
DSSTOX Substance ID |
DTXSID20163883 | |
Record name | Fluorocyclopentane | |
Source | EPA DSSTox | |
URL | https://comptox.epa.gov/dashboard/DTXSID20163883 | |
Description | DSSTox provides a high quality public chemistry resource for supporting improved predictive toxicology. | |
Molecular Weight |
88.12 g/mol | |
Source | PubChem | |
URL | https://pubchem.ncbi.nlm.nih.gov | |
Description | Data deposited in or computed by PubChem | |
CAS No. |
1481-36-3 | |
Record name | Fluorocyclopentane | |
Source | ChemIDplus | |
URL | https://pubchem.ncbi.nlm.nih.gov/substance/?source=chemidplus&sourceid=0001481363 | |
Description | ChemIDplus is a free, web search system that provides access to the structure and nomenclature authority files used for the identification of chemical substances cited in National Library of Medicine (NLM) databases, including the TOXNET system. | |
Record name | Fluorocyclopentane | |
Source | EPA DSSTox | |
URL | https://comptox.epa.gov/dashboard/DTXSID20163883 | |
Description | DSSTox provides a high quality public chemistry resource for supporting improved predictive toxicology. | |
Record name | Fluorocyclopentane | |
Source | European Chemicals Agency (ECHA) | |
URL | https://echa.europa.eu/information-on-chemicals | |
Description | The European Chemicals Agency (ECHA) is an agency of the European Union which is the driving force among regulatory authorities in implementing the EU's groundbreaking chemicals legislation for the benefit of human health and the environment as well as for innovation and competitiveness. | |
Explanation | Use of the information, documents and data from the ECHA website is subject to the terms and conditions of this Legal Notice, and subject to other binding limitations provided for under applicable law, the information, documents and data made available on the ECHA website may be reproduced, distributed and/or used, totally or in part, for non-commercial purposes provided that ECHA is acknowledged as the source: "Source: European Chemicals Agency, http://echa.europa.eu/". Such acknowledgement must be included in each copy of the material. ECHA permits and encourages organisations and individuals to create links to the ECHA website under the following cumulative conditions: Links can only be made to webpages that provide a link to the Legal Notice page. | |
Retrosynthesis Analysis
AI-Powered Synthesis Planning: Our tool employs the Template_relevance Pistachio, Template_relevance Bkms_metabolic, Template_relevance Pistachio_ringbreaker, Template_relevance Reaxys, Template_relevance Reaxys_biocatalysis model, leveraging a vast database of chemical reactions to predict feasible synthetic routes.
One-Step Synthesis Focus: Specifically designed for one-step synthesis, it provides concise and direct routes for your target compounds, streamlining the synthesis process.
Accurate Predictions: Utilizing the extensive PISTACHIO, BKMS_METABOLIC, PISTACHIO_RINGBREAKER, REAXYS, REAXYS_BIOCATALYSIS database, our tool offers high-accuracy predictions, reflecting the latest in chemical research and data.
Strategy Settings
Precursor scoring | Relevance Heuristic |
---|---|
Min. plausibility | 0.01 |
Model | Template_relevance |
Template Set | Pistachio/Bkms_metabolic/Pistachio_ringbreaker/Reaxys/Reaxys_biocatalysis |
Top-N result to add to graph | 6 |
Feasible Synthetic Routes
Disclaimer and Information on In-Vitro Research Products
Please be aware that all articles and product information presented on BenchChem are intended solely for informational purposes. The products available for purchase on BenchChem are specifically designed for in-vitro studies, which are conducted outside of living organisms. In-vitro studies, derived from the Latin term "in glass," involve experiments performed in controlled laboratory settings using cells or tissues. It is important to note that these products are not categorized as medicines or drugs, and they have not received approval from the FDA for the prevention, treatment, or cure of any medical condition, ailment, or disease. We must emphasize that any form of bodily introduction of these products into humans or animals is strictly prohibited by law. It is essential to adhere to these guidelines to ensure compliance with legal and ethical standards in research and experimentation.