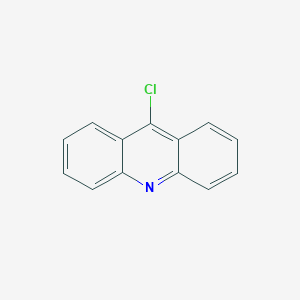
9-Chloroacridine
Overview
Description
9-Chloroacridine (C₁₃H₈ClN) is a heterocyclic aromatic compound featuring a chlorine atom at the 9-position of the acridine scaffold. This structural motif confers significant reactivity, making it a versatile intermediate in medicinal chemistry and materials science. Its synthesis typically involves Friedel-Crafts cyclization of diarylamine precursors using POCl₃ , or catalytic methods employing metal-organic frameworks (MOFs) like Cu₃(BTC)₂ . A key challenge in handling this compound is its susceptibility to hydrolysis, which generates 9-acridone impurities under neutral or acidic conditions . Despite this, its role as a precursor to bioactive derivatives (e.g., 9-aminoacridines, amsacrine) underscores its importance in drug discovery .
Preparation Methods
Classical Synthesis via N-Phenylanthranilic Acid or Acridone
Reaction Mechanism and Substrate Selection
The classical preparation of 9-chloroacridine involves cyclodehydration of N-phenylanthranilic acid (C₁₃H₁₁NO₂) or acridone (C₁₃H₉NO) using POCl₃ as both a solvent and dehydrating agent . The reaction proceeds via a Friedel-Crafts mechanism, where POCl₃ activates the carbonyl group of the substrate, facilitating intramolecular cyclization to form the acridine core. N-Phenylanthranilic acid is preferred for its commercial availability, while acridone offers higher purity due to reduced side reactions .
Standard Procedure and Optimization
In a representative protocol, 50 g (0.23 mol) of N-phenylanthranilic acid is mixed with 160 mL (1.76 mol) of freshly distilled POCl₃ in a round-bottom flask . The mixture is heated to 85–90°C on a water bath, triggering a vigorous exothermic reaction. After subsidence, the temperature is raised to 135–140°C for 2 hours to ensure complete cyclization. Excess POCl₃ is removed under vacuum (50 mm Hg), and the residue is quenched in a cold ammonia-chloroform mixture. The crude product is isolated by solvent extraction, yielding 50 g (theoretical) of this compound with a melting point of 117–118°C .
Critical Notes:
-
POCl₃ Purity: Distilled POCl₃ is essential to prevent hydrolysis by residual water, which generates phosphoric acid and compromises yield .
-
Quenching Protocol: Rapid neutralization with ammonia minimizes hydrolysis of this compound to 9-acridone, a common side reaction .
Modified Procedures for Enhanced Efficiency
Hexane Extraction Method
A streamlined protocol from the Royal Society of Chemistry replaces chloroform with n-hexane for improved extraction efficiency . Here, 2.3 g (11 mmol) of N-phenylanthranilic acid is reacted with 5 mL (50 mmol) of POCl₃ at 120°C for 1.5 hours. After quenching, the mixture is stirred with 300 mL of n-hexane for 12 hours, allowing this compound to partition into the organic phase. Evaporation yields 2.2 g (95%) of product, demonstrating superior scalability compared to classical methods .
Recrystallization for High Purity
For applications requiring ultrapure this compound, the crude product is dissolved in hot ethanol and treated with 0.5% ammonia until turbid. Decolorization with activated carbon followed by rapid cooling yields white crystals (m.p. 119–120°C) with >99% purity . This step eliminates residual phosphorus byproducts, which are common in large-scale syntheses.
Modern Parallel Synthesis Strategies
Ullmann Coupling and Friedel-Crafts Cyclization
Recent advances employ Ullmann coupling of 2-bromoaryl carboxylic acids with anilines to generate diarylamine intermediates, which undergo Friedel-Crafts cyclization with POCl₃ . For example, methyl salicylate derivatives are converted to aryl triflates, coupled with substituted anilines, and cyclized under POCl₃ reflux (120°C, 3 hours). This method achieves 95% yield and enables modular substitution on the acridine ring .
High-Throughput Amination
In drug discovery pipelines, this compound is activated as 9-phenoxyacridine by treatment with phenol and cesium carbonate in DMSO . Subsequent reaction with diamines (e.g., 1,3-diaminopropane) at 100°C for 4 hours produces 9-aminoacridine derivatives via nucleophilic aromatic substitution. This approach supports parallel synthesis of 48 compounds simultaneously, with purities >90% after solid-phase extraction .
Comparative Analysis of Preparation Methods
Industrial and Pharmaceutical Applications
Antimalarial Drug Precursors
This compound is a key intermediate in synthesizing quinacrine analogues, which inhibit hemozoin crystallization in Plasmodium parasites . Modern parallel methods enable rapid optimization of side chains for enhanced bioavailability and reduced toxicity.
Fluorescent Materials
The chloro group in this compound serves as a leaving group for Suzuki-Miyaura cross-coupling, producing acridine-based fluorophores with tunable emission wavelengths (450–600 nm) . These materials are integral to OLED technologies and bioimaging probes.
Chemical Reactions Analysis
3.1. Redox Reactions
The electrochemical behavior of 9-chloroacridine has been extensively studied. It undergoes redox reactions characterized by:
- Oxidation : The oxidation process begins with the formation of a cation radical monomer, which can further dimerize or oxidize to form new cation radicals. This process is pH-dependent and diffusion-controlled .
- Reduction : Reduction leads to the formation of radical monomers that can stabilize through dimerization. The interaction with double-stranded DNA has been shown to form stable complexes through intercalation, which is significant for drug design .
Table 2: Electrochemical Behavior of this compound
Reaction Type | Process Description | Observations |
---|---|---|
Oxidation | Formation of cation radicals | Dimerization occurs |
Reduction | Formation of radical monomers | Stabilization via dimerization |
3.2. Nucleophilic Substitution Reactions
Nucleophilic substitution reactions are predominant at the 9-position due to lower electron density compared to other positions on the acridine ring:
- Nucleophilic Attack : Nucleophiles preferentially attack at the nitrogen atom or the carbon at position 9, leading to various derivatives such as amino-acridines .
Table 3: Nucleophilic Substitution Reactions
Nucleophile | Product | Reaction Conditions |
---|---|---|
Amines | 9-Aminoacridines | Basic conditions |
Alcohols | Alkoxy derivatives | Reflux in alcohol solvent |
Scientific Research Applications
Medicinal Chemistry Applications
1.1 Anti-Cancer Activity
9-Chloroacridine and its derivatives have been extensively studied for their anti-cancer properties. Research indicates that acridine compounds, including this compound, exhibit significant cytotoxic effects against various cancer cell lines.
- Mechanism of Action : The compound acts primarily through DNA intercalation, which disrupts the replication and transcription processes in cancer cells. This leads to apoptosis, particularly in leukemia and solid tumor cell lines .
- Case Study : A study demonstrated that 2-methyl-9-chloroacridine derivatives showed potent anti-proliferative activity against A-549 (human lung carcinoma) and MCF-7 (breast cancer) cell lines, with IC50 values indicating strong cytotoxicity .
Table 1: Anti-Cancer Efficacy of this compound Derivatives
Compound | Cell Line | IC50 (µM) | Mechanism of Action |
---|---|---|---|
2-Methyl-9-chloroacridine | A-549 | 0.90 | DNA intercalation |
9-Anilino acridine derivative | MCF-7 | 0.75 | Apoptosis induction |
LS-1-10 | Colon cancer | 0.50 | Autophagy inhibition |
1.2 Antimicrobial Properties
In addition to its anti-cancer applications, this compound exhibits antimicrobial activity against various pathogens.
- Study Findings : Research has shown that acridine derivatives can act as effective antibacterial agents. For instance, a derivative was tested for its efficacy against resistant strains of bacteria, demonstrating notable antibacterial properties .
Analytical Chemistry Applications
2.1 Chromogenic Reagent
This compound serves as a chromogenic reagent in analytical chemistry, particularly for the detection of certain compounds.
- Tetracycline Detection : A visible spectrophotometric method using this compound has been developed for the detection of tetracycline hydrochloride in pharmaceutical formulations. This method is noted for its simplicity and sensitivity, allowing for accurate quantification of tetracycline levels in various samples .
Table 2: Analytical Applications of this compound
Application | Methodology | Target Compound |
---|---|---|
Tetracycline Detection | Spectrophotometry | Tetracycline hydrochloride |
Paracetamol Quantification | Colorimetric Determination | Paracetamol |
Primary Aromatic Amines Detection | Improved Colorimetric Method | Local Anesthetics |
Photochemical Applications
3.1 Photochemical Reactions
The photochemical properties of this compound have been investigated, revealing its potential use in photodynamic therapy (PDT) for cancer treatment.
Mechanism of Action
The mechanism of action of 9-Chloroacridine involves its ability to intercalate into DNA, disrupting the normal function of the DNA molecule. This intercalation can inhibit the activity of enzymes such as topoisomerases and telomerases, leading to the inhibition of cell proliferation. Additionally, it can interact with various molecular targets through hydrogen bonding and hydrophobic interactions .
Comparison with Similar Compounds
Comparative Analysis with Similar Compounds
Reactivity and Functionalization
The chlorine atom at the 9-position enables diverse nucleophilic substitutions:
- With Amines: Forms 9-aminoacridines, which exhibit enhanced basicity (pKa ~10 vs. acridine’s pKa ~5) and anticancer activity .
- With Azides : Generates 9-azidoacridine for click chemistry applications .
- With Phenols/Thiols: Produces 9-phenoxyacridines or 9-thioacridines, explored for anti-inflammatory properties .
Comparison of Derivatives :
- Key Insight: 9-Chloroacridine-derived compounds exhibit nanomolar to micromolar potency, with amsacrine and aminoacridines showing superior efficacy but variable toxicity profiles .
Catalytic and Electronic Effects
In catalysis, this compound’s electron-withdrawing chlorine substituent reduces ligand efficacy compared to electron-rich analogs:
Biological Activity
9-Chloroacridine, a derivative of acridine, has garnered attention in the field of medicinal chemistry due to its diverse biological activities, particularly its potential as an anticancer agent. This article synthesizes recent research findings regarding the biological activity of this compound, focusing on its anticancer properties, mechanisms of action, and other relevant biological effects.
This compound is characterized by the presence of a chlorine atom at the 9-position of the acridine ring system. This modification enhances its interaction with biological targets, primarily DNA, leading to various biological activities.
Research indicates that this compound and its derivatives exhibit significant anticancer activity through several mechanisms:
- DNA Intercalation : Compounds like this compound are known to intercalate into DNA, disrupting replication and transcription processes. This action leads to increased DNA damage and apoptosis in cancer cells .
- Cytotoxicity : Studies have shown that this compound derivatives demonstrate cytotoxic effects against various cancer cell lines, including A549 (lung carcinoma) and MCF-7 (breast cancer) cells. For instance, a derivative exhibited a value of 187.5 µg/mL against A549 cells .
- Inhibition of Glycolysis : Some studies suggest that these compounds can inhibit aerobic glycolysis in cancer cells, further contributing to their antiproliferative effects .
Case Studies
- Cytotoxicity Against Melanoma : A study evaluated the cytotoxic effects of 9-chloro-1-nitroacridine and its derivatives against melanotic and amelanotic melanoma forms. The results indicated that these compounds could significantly inhibit cell proliferation and induce apoptosis through energy metabolism disruption .
- Synthesis and Evaluation : A series of novel acridinyl amino acid derivatives were synthesized based on this compound. These derivatives showed improved antitumor activity compared to traditional chemotherapeutics like amsacrine. The study highlighted that the antiproliferative activity depended on the specific amino acid modifications made to the acridine structure .
Comparative Biological Activity
The following table summarizes key findings on the biological activity of this compound derivatives compared to other known anticancer agents:
Compound | Cell Line Tested | CTC50 (µg/mL) | Mechanism of Action |
---|---|---|---|
This compound | A549 | 187.5 | DNA intercalation, glycolysis inhibition |
9-Chloro-1-nitroacridine | Melanoma | Not specified | Apoptosis induction |
Amsacrine | Various | ~200 | DNA intercalation |
Nitracrine | Various | ~150 | DNA intercalation |
Other Biological Activities
In addition to anticancer properties, this compound has been explored for other biological activities:
- Antimicrobial Activity : Recent studies have shown that derivatives of this compound possess antibacterial properties against both Gram-positive and Gram-negative bacteria .
- Larvicidal Activity : Some synthesized analogs have been tested for their effectiveness against mosquito larvae, indicating potential use in vector control strategies .
Q & A
Basic Research Questions
Q. What are the established synthetic routes for 9-chloroacridine, and how do reaction conditions influence yield?
- This compound is synthesized via cyclization of diphenylamine-2-carboxylic acid (phenylanthranilic acid) using phosphoryl chloride (POCl₃). Alternative methods include Ullmann condensation of aniline with o-chlorobenzoic acid followed by POCl₃-mediated cyclization . Reaction temperature (e.g., reflux conditions) and stoichiometric ratios of POCl₃ are critical for optimizing yields, which typically range from 70–85% under controlled conditions.
Q. How is this compound utilized in spectrophotometric drug analysis?
- This compound reacts with nucleophilic groups in drugs like aspirin and pyridoxine hydrochloride via nucleophilic substitution, forming colored complexes detectable at 532–534 nm. Key parameters include:
Parameter | Aspirin | Pyridoxine |
---|---|---|
Linear range (µg/mL) | 0.2–18 | 0.4–24 |
Molar absorptivity (L·mol⁻¹·cm⁻¹) | 1.1×10⁴ | 7×10³ |
Recovery rate (%) | 99.87 | 100.00 |
This method is validated for pharmaceutical formulations with minimal interference from excipients . |
Q. What are the primary applications of this compound in biological research?
- This compound derivatives are studied for DNA intercalation and electrochemical interactions, particularly in cancer research. For example, this compound forms stable adducts with DNA via π-π stacking, which can be quantified using cyclic voltammetry at glassy carbon electrodes .
Advanced Research Questions
Q. How can researchers address discrepancies in reported stability constants of this compound-drug complexes?
- Stability constants (e.g., 6.38×10⁴ L/mol for aspirin vs. 9.25×10⁴ L/mol for pyridoxine) vary due to solvent polarity, pH, and temperature. To resolve contradictions:
- Validate buffer systems (e.g., acetate buffer pH 4.0 vs. phosphate buffer pH 7.4).
- Use isothermal titration calorimetry (ITC) to corroborate spectrophotometric data .
Q. What strategies mitigate contamination by acridone in this compound samples?
- Acridone (a common byproduct at ~0.3%) arises from hydrolysis of this compound. Mitigation approaches include:
- Purification via column chromatography (silica gel, chloroform:methanol 9:1).
- Storage under anhydrous conditions (e.g., desiccated argon atmosphere) .
Q. How can this compound be functionalized for targeted drug delivery systems?
- Coupling with amines (e.g., aromatic amines, hydroxylamines) via nucleophilic aromatic substitution introduces functional groups. Example:
- React this compound with O-phenylhydroxylamine (catalyzed by Pd) to yield DNA-targeting N-(9’-acridinyl) derivatives .
Q. What experimental design considerations are critical for electrochemical studies of this compound-DNA interactions?
- Key factors include:
- Electrode pretreatment (polishing with alumina slurry for reproducibility).
- Control of scan rate (0.1–1.0 V/s) to distinguish diffusion-controlled vs. adsorption-driven processes.
- Use of differential pulse voltammetry (DPV) for enhanced sensitivity in detecting oxidation peaks .
Q. Methodological Guidance
Q. How to optimize a spectrophotometric assay using this compound for novel compounds?
- Step 1: Screen reaction pH (2.0–7.0) and temperature (25–60°C) to maximize complex formation.
- Step 2: Validate linearity using ≥6 calibration points and assess precision via intraday/interday RSD (<2%).
- Step 3: Perform interference studies with common excipients (e.g., lactose, starch) .
Q. How to formulate research questions on this compound’s mechanism of action using FINER criteria?
- Feasible: "Does this compound exhibit pH-dependent binding to double-stranded DNA?"
- Novel: "How do substituents at the acridine C9 position alter intercalation kinetics?"
- Relevant: Align with therapeutic goals (e.g., antimalarial or anticancer drug design) .
Q. Data Contradiction Analysis
- Case Study: Conflicting reports on this compound’s molar absorptivity in drug assays.
- Resolution: Verify instrument calibration (e.g., wavelength accuracy ±1 nm) and reagent purity (HPLC-grade solvents). Replicate experiments under standardized conditions .
Properties
IUPAC Name |
9-chloroacridine | |
---|---|---|
Source | PubChem | |
URL | https://pubchem.ncbi.nlm.nih.gov | |
Description | Data deposited in or computed by PubChem | |
InChI |
InChI=1S/C13H8ClN/c14-13-9-5-1-3-7-11(9)15-12-8-4-2-6-10(12)13/h1-8H | |
Source | PubChem | |
URL | https://pubchem.ncbi.nlm.nih.gov | |
Description | Data deposited in or computed by PubChem | |
InChI Key |
BPXINCHFOLVVSG-UHFFFAOYSA-N | |
Source | PubChem | |
URL | https://pubchem.ncbi.nlm.nih.gov | |
Description | Data deposited in or computed by PubChem | |
Canonical SMILES |
C1=CC=C2C(=C1)C(=C3C=CC=CC3=N2)Cl | |
Source | PubChem | |
URL | https://pubchem.ncbi.nlm.nih.gov | |
Description | Data deposited in or computed by PubChem | |
Molecular Formula |
C13H8ClN | |
Source | PubChem | |
URL | https://pubchem.ncbi.nlm.nih.gov | |
Description | Data deposited in or computed by PubChem | |
DSSTOX Substance ID |
DTXSID4061622 | |
Record name | Acridine, 9-chloro- | |
Source | EPA DSSTox | |
URL | https://comptox.epa.gov/dashboard/DTXSID4061622 | |
Description | DSSTox provides a high quality public chemistry resource for supporting improved predictive toxicology. | |
Molecular Weight |
213.66 g/mol | |
Source | PubChem | |
URL | https://pubchem.ncbi.nlm.nih.gov | |
Description | Data deposited in or computed by PubChem | |
CAS No. |
1207-69-8 | |
Record name | 9-Chloroacridine | |
Source | CAS Common Chemistry | |
URL | https://commonchemistry.cas.org/detail?cas_rn=1207-69-8 | |
Description | CAS Common Chemistry is an open community resource for accessing chemical information. Nearly 500,000 chemical substances from CAS REGISTRY cover areas of community interest, including common and frequently regulated chemicals, and those relevant to high school and undergraduate chemistry classes. This chemical information, curated by our expert scientists, is provided in alignment with our mission as a division of the American Chemical Society. | |
Explanation | The data from CAS Common Chemistry is provided under a CC-BY-NC 4.0 license, unless otherwise stated. | |
Record name | 9-Chloroacridine | |
Source | ChemIDplus | |
URL | https://pubchem.ncbi.nlm.nih.gov/substance/?source=chemidplus&sourceid=0001207698 | |
Description | ChemIDplus is a free, web search system that provides access to the structure and nomenclature authority files used for the identification of chemical substances cited in National Library of Medicine (NLM) databases, including the TOXNET system. | |
Record name | 9-Chloroacridine | |
Source | DTP/NCI | |
URL | https://dtp.cancer.gov/dtpstandard/servlet/dwindex?searchtype=NSC&outputformat=html&searchlist=51950 | |
Description | The NCI Development Therapeutics Program (DTP) provides services and resources to the academic and private-sector research communities worldwide to facilitate the discovery and development of new cancer therapeutic agents. | |
Explanation | Unless otherwise indicated, all text within NCI products is free of copyright and may be reused without our permission. Credit the National Cancer Institute as the source. | |
Record name | Acridine, 9-chloro- | |
Source | EPA Chemicals under the TSCA | |
URL | https://www.epa.gov/chemicals-under-tsca | |
Description | EPA Chemicals under the Toxic Substances Control Act (TSCA) collection contains information on chemicals and their regulations under TSCA, including non-confidential content from the TSCA Chemical Substance Inventory and Chemical Data Reporting. | |
Record name | Acridine, 9-chloro- | |
Source | EPA DSSTox | |
URL | https://comptox.epa.gov/dashboard/DTXSID4061622 | |
Description | DSSTox provides a high quality public chemistry resource for supporting improved predictive toxicology. | |
Record name | 9-chloroacridine | |
Source | European Chemicals Agency (ECHA) | |
URL | https://echa.europa.eu/substance-information/-/substanceinfo/100.013.541 | |
Description | The European Chemicals Agency (ECHA) is an agency of the European Union which is the driving force among regulatory authorities in implementing the EU's groundbreaking chemicals legislation for the benefit of human health and the environment as well as for innovation and competitiveness. | |
Explanation | Use of the information, documents and data from the ECHA website is subject to the terms and conditions of this Legal Notice, and subject to other binding limitations provided for under applicable law, the information, documents and data made available on the ECHA website may be reproduced, distributed and/or used, totally or in part, for non-commercial purposes provided that ECHA is acknowledged as the source: "Source: European Chemicals Agency, http://echa.europa.eu/". Such acknowledgement must be included in each copy of the material. ECHA permits and encourages organisations and individuals to create links to the ECHA website under the following cumulative conditions: Links can only be made to webpages that provide a link to the Legal Notice page. | |
Synthesis routes and methods
Procedure details
Retrosynthesis Analysis
AI-Powered Synthesis Planning: Our tool employs the Template_relevance Pistachio, Template_relevance Bkms_metabolic, Template_relevance Pistachio_ringbreaker, Template_relevance Reaxys, Template_relevance Reaxys_biocatalysis model, leveraging a vast database of chemical reactions to predict feasible synthetic routes.
One-Step Synthesis Focus: Specifically designed for one-step synthesis, it provides concise and direct routes for your target compounds, streamlining the synthesis process.
Accurate Predictions: Utilizing the extensive PISTACHIO, BKMS_METABOLIC, PISTACHIO_RINGBREAKER, REAXYS, REAXYS_BIOCATALYSIS database, our tool offers high-accuracy predictions, reflecting the latest in chemical research and data.
Strategy Settings
Precursor scoring | Relevance Heuristic |
---|---|
Min. plausibility | 0.01 |
Model | Template_relevance |
Template Set | Pistachio/Bkms_metabolic/Pistachio_ringbreaker/Reaxys/Reaxys_biocatalysis |
Top-N result to add to graph | 6 |
Feasible Synthetic Routes
Disclaimer and Information on In-Vitro Research Products
Please be aware that all articles and product information presented on BenchChem are intended solely for informational purposes. The products available for purchase on BenchChem are specifically designed for in-vitro studies, which are conducted outside of living organisms. In-vitro studies, derived from the Latin term "in glass," involve experiments performed in controlled laboratory settings using cells or tissues. It is important to note that these products are not categorized as medicines or drugs, and they have not received approval from the FDA for the prevention, treatment, or cure of any medical condition, ailment, or disease. We must emphasize that any form of bodily introduction of these products into humans or animals is strictly prohibited by law. It is essential to adhere to these guidelines to ensure compliance with legal and ethical standards in research and experimentation.