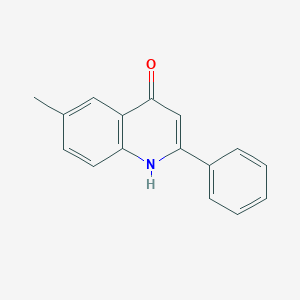
4-Hydroxy-6-methyl-2-phenylquinoline
Overview
Description
4-Hydroxy-6-methyl-2-phenylquinoline is a quinoline derivative with the molecular formula C16H13NO. This compound is characterized by a hydroxyl group at the fourth position, a methyl group at the sixth position, and a phenyl group at the second position of the quinoline ring. Quinoline derivatives are known for their diverse biological activities and applications in various fields, including medicinal chemistry and materials science .
Scientific Research Applications
4-Hydroxy-6-methyl-2-phenylquinoline has a wide range of applications in scientific research:
Chemistry: Used as a building block for the synthesis of more complex quinoline derivatives.
Biology: Investigated for its potential antimicrobial and anticancer properties.
Medicine: Explored as a lead compound for the development of new therapeutic agents.
Industry: Utilized in the production of dyes, pigments, and other materials.
Mechanism of Action
Target of Action
4-Hydroxy-6-methyl-2-phenylquinoline is a derivative of quinoline, a nitrogen-based heterocyclic aromatic compound . Quinoline and its derivatives are known to exhibit a broad spectrum of bio-responses, including anticancer, antioxidant, anti-inflammatory, antimalarial, anti-SARS-CoV-2, and antituberculosis activities . .
Mode of Action
Quinoline derivatives are known to interact with various receptors through a variety of known and unknown mechanisms, including epigenetic modification . They differ from classic toxins in several ways such as low-dose effect, non-monotonic dose, and trans-generational effects .
Biochemical Pathways
Quinoline derivatives are known to influence a wide range of biological activities and are still being applied to create compounds with wide-ranging pharmacological activities .
Result of Action
Quinoline derivatives are known to have a broad range of biological activities, including anticancer, antioxidant, anti-inflammatory, antimalarial, anti-sars-cov-2, and antituberculosis activities .
Safety and Hazards
The compound is classified as having Acute Toxicity 4 Oral, Aquatic Chronic 4, and Eye Damage 1 according to the Globally Harmonized System of Classification and Labelling of Chemicals (GHS). The safety precautions include avoiding eye contact and ingestion, and it should be handled and stored as a combustible solid .
Future Directions
Quinoline and its derivatives, including “4-Hydroxy-6-methyl-2-phenylquinoline”, have been the focus of many research studies due to their wide range of biological and pharmacological activities . Future research may focus on developing new synthetic methods and investigating new structural prototypes with more effective antimalarial, antimicrobial, and anticancer activity .
Preparation Methods
Synthetic Routes and Reaction Conditions: The synthesis of 4-Hydroxy-6-methyl-2-phenylquinoline typically involves the cyclization of appropriate precursors under specific conditions. One common method is the Friedländer synthesis, which involves the condensation of aniline derivatives with carbonyl compounds in the presence of acidic or basic catalysts . Another method is the Skraup synthesis, which uses glycerol, aniline, and sulfuric acid as reactants .
Industrial Production Methods: Industrial production of this compound may involve large-scale batch or continuous processes, utilizing optimized reaction conditions to maximize yield and purity. Catalysts such as Lewis acids or transition metal complexes can be employed to enhance reaction efficiency .
Chemical Reactions Analysis
Types of Reactions: 4-Hydroxy-6-methyl-2-phenylquinoline undergoes various chemical reactions, including:
Oxidation: The hydroxyl group can be oxidized to form quinone derivatives.
Reduction: The compound can be reduced to form dihydroquinoline derivatives.
Substitution: Electrophilic substitution reactions can occur at the aromatic ring, leading to the formation of various substituted derivatives.
Common Reagents and Conditions:
Oxidation: Reagents such as potassium permanganate or chromium trioxide in acidic conditions.
Reduction: Catalytic hydrogenation using palladium on carbon or sodium borohydride.
Substitution: Halogenation using bromine or chlorination using chlorine gas.
Major Products Formed:
Oxidation: Quinone derivatives.
Reduction: Dihydroquinoline derivatives.
Substitution: Halogenated quinoline derivatives.
Comparison with Similar Compounds
- 4-Hydroxy-7-methoxy-2-phenylquinoline
- 4-Hydroxy-8-methyl-2-propylquinoline
- 8-Chloro-4-hydroxy-2-phenylquinoline
Comparison: 4-Hydroxy-6-methyl-2-phenylquinoline is unique due to its specific substitution pattern, which imparts distinct chemical and biological properties. Compared to its analogs, it may exhibit different reactivity and potency in various applications .
Properties
IUPAC Name |
6-methyl-2-phenyl-1H-quinolin-4-one | |
---|---|---|
Source | PubChem | |
URL | https://pubchem.ncbi.nlm.nih.gov | |
Description | Data deposited in or computed by PubChem | |
InChI |
InChI=1S/C16H13NO/c1-11-7-8-14-13(9-11)16(18)10-15(17-14)12-5-3-2-4-6-12/h2-10H,1H3,(H,17,18) | |
Source | PubChem | |
URL | https://pubchem.ncbi.nlm.nih.gov | |
Description | Data deposited in or computed by PubChem | |
InChI Key |
SWRQHHJAXCNFGU-UHFFFAOYSA-N | |
Source | PubChem | |
URL | https://pubchem.ncbi.nlm.nih.gov | |
Description | Data deposited in or computed by PubChem | |
Canonical SMILES |
CC1=CC2=C(C=C1)NC(=CC2=O)C3=CC=CC=C3 | |
Source | PubChem | |
URL | https://pubchem.ncbi.nlm.nih.gov | |
Description | Data deposited in or computed by PubChem | |
Molecular Formula |
C16H13NO | |
Source | PubChem | |
URL | https://pubchem.ncbi.nlm.nih.gov | |
Description | Data deposited in or computed by PubChem | |
DSSTOX Substance ID |
DTXSID10352648 | |
Record name | 4-Hydroxy-6-methyl-2-phenylquinoline | |
Source | EPA DSSTox | |
URL | https://comptox.epa.gov/dashboard/DTXSID10352648 | |
Description | DSSTox provides a high quality public chemistry resource for supporting improved predictive toxicology. | |
Molecular Weight |
235.28 g/mol | |
Source | PubChem | |
URL | https://pubchem.ncbi.nlm.nih.gov | |
Description | Data deposited in or computed by PubChem | |
CAS No. |
1148-49-8 | |
Record name | 4-Hydroxy-6-methyl-2-phenylquinoline | |
Source | EPA DSSTox | |
URL | https://comptox.epa.gov/dashboard/DTXSID10352648 | |
Description | DSSTox provides a high quality public chemistry resource for supporting improved predictive toxicology. | |
Record name | 1148-49-8 | |
Source | European Chemicals Agency (ECHA) | |
URL | https://echa.europa.eu/information-on-chemicals | |
Description | The European Chemicals Agency (ECHA) is an agency of the European Union which is the driving force among regulatory authorities in implementing the EU's groundbreaking chemicals legislation for the benefit of human health and the environment as well as for innovation and competitiveness. | |
Explanation | Use of the information, documents and data from the ECHA website is subject to the terms and conditions of this Legal Notice, and subject to other binding limitations provided for under applicable law, the information, documents and data made available on the ECHA website may be reproduced, distributed and/or used, totally or in part, for non-commercial purposes provided that ECHA is acknowledged as the source: "Source: European Chemicals Agency, http://echa.europa.eu/". Such acknowledgement must be included in each copy of the material. ECHA permits and encourages organisations and individuals to create links to the ECHA website under the following cumulative conditions: Links can only be made to webpages that provide a link to the Legal Notice page. | |
Synthesis routes and methods
Procedure details
Disclaimer and Information on In-Vitro Research Products
Please be aware that all articles and product information presented on BenchChem are intended solely for informational purposes. The products available for purchase on BenchChem are specifically designed for in-vitro studies, which are conducted outside of living organisms. In-vitro studies, derived from the Latin term "in glass," involve experiments performed in controlled laboratory settings using cells or tissues. It is important to note that these products are not categorized as medicines or drugs, and they have not received approval from the FDA for the prevention, treatment, or cure of any medical condition, ailment, or disease. We must emphasize that any form of bodily introduction of these products into humans or animals is strictly prohibited by law. It is essential to adhere to these guidelines to ensure compliance with legal and ethical standards in research and experimentation.