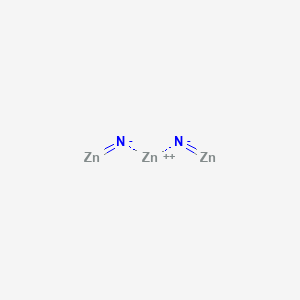
Zinc nitride
Overview
Description
Zinc nitride is an inorganic compound composed of zinc and nitrogen, typically obtained as blue-gray cubic crystals. It is a semiconductor with a cubic anti-bixbyite structure. The compound has a molar mass of 224.154 grams per mole and a density of 6.22 grams per cubic centimeter. It decomposes at temperatures around 700°C and is insoluble in water .
Synthetic Routes and Reaction Conditions:
Thermal Decomposition of Zincamide: this compound can be synthesized by thermally decomposing zincamide (zinc diamine) in an anaerobic environment at temperatures exceeding 200°C.
Heating Zinc in Ammonia: Another method involves heating zinc to 600°C in a current of ammonia, producing this compound and hydrogen gas as a by-product. [ 3 \text{Zn} + 2 \text{NH}_3 \rightarrow \text{Zn}_3\text{N}_2 + 3 \text{H}_2 ]
Electric Discharge: this compound can also be formed by producing an electric discharge between zinc electrodes in a nitrogen atmosphere.
Chemical Vapor Deposition: Thin films of this compound can be produced by chemical vapor deposition of bis(trimethylsilyl)amidozinc with ammonia gas onto silica or zinc oxide-coated alumina at temperatures between 275 to 410°C.
Industrial Production Methods:
- The industrial production of this compound typically involves the chemical vapor deposition method due to its efficiency in producing high-purity thin films suitable for various applications.
Types of Reactions:
Oxidation: this compound reacts violently with water to form ammonia and zinc oxide. [ \text{Zn}_3\text{N}_2 + 3 \text{H}_2\text{O} \rightarrow 3 \text{ZnO} + 2 \text{NH}_3 ]
Reaction with Lithium: this compound reacts with lithium (produced in an electrochemical cell) by insertion, initially converting into lithium zinc in a matrix of beta-lithium nitride.
Common Reagents and Conditions:
Water: Reacts with this compound to produce ammonia and zinc oxide.
Ammonia: Used in the synthesis of this compound through heating with zinc.
Lithium: Used in electrochemical reactions with this compound.
Major Products:
Ammonia and Zinc Oxide: Formed from the reaction of this compound with water.
Lithium this compound and Metallic Zinc: Formed from the reaction of this compound with lithium.
Scientific Research Applications
Zinc nitride has a wide range of applications in scientific research due to its unique properties:
Chemistry: Used in the synthesis of various compounds and as a precursor for other nitride materials.
Mechanism of Action
The mechanism by which zinc nitride exerts its effects is primarily through its semiconductor properties. It has a reported bandgap of approximately 3.2 electron volts, making it suitable for applications in optoelectronics. The compound’s high electron mobility and ability to form thin films with high carrier concentrations make it an excellent material for use in electronic devices .
Comparison with Similar Compounds
Zinc Oxide (ZnO): A widely used semiconductor with applications in sensors, transparent conductive oxides, and solar cells. Unlike zinc nitride, zinc oxide is soluble in water and has a different crystal structure.
Manganese(III) Oxide (Mn2O3): Shares a similar anti-bixbyite crystal structure with this compound but has different chemical properties and applications.
Gallium Nitride (GaN): Another semiconductor used in light-emitting diodes and high-frequency transistors. Gallium nitride has a wider bandgap compared to this compound, making it suitable for different applications.
Uniqueness of this compound:
- This compound’s unique combination of high electron mobility, suitable bandgap, and ability to form high-purity thin films distinguishes it from other similar compounds. Its applications in both optoelectronics and as a probe for metal ion detection highlight its versatility and potential for future research and industrial applications.
Properties
IUPAC Name |
zinc;azanidylidenezinc | |
---|---|---|
Source | PubChem | |
URL | https://pubchem.ncbi.nlm.nih.gov | |
Description | Data deposited in or computed by PubChem | |
InChI |
InChI=1S/2N.3Zn/q2*-1;;;+2 | |
Source | PubChem | |
URL | https://pubchem.ncbi.nlm.nih.gov | |
Description | Data deposited in or computed by PubChem | |
InChI Key |
AKJVMGQSGCSQBU-UHFFFAOYSA-N | |
Source | PubChem | |
URL | https://pubchem.ncbi.nlm.nih.gov | |
Description | Data deposited in or computed by PubChem | |
Canonical SMILES |
[N-]=[Zn].[N-]=[Zn].[Zn+2] | |
Source | PubChem | |
URL | https://pubchem.ncbi.nlm.nih.gov | |
Description | Data deposited in or computed by PubChem | |
Molecular Formula |
Zn3N2, N2Zn3 | |
Record name | zinc nitride | |
Source | Wikipedia | |
URL | https://en.wikipedia.org/wiki/Zinc_nitride | |
Description | Chemical information link to Wikipedia. | |
Source | PubChem | |
URL | https://pubchem.ncbi.nlm.nih.gov | |
Description | Data deposited in or computed by PubChem | |
Molecular Weight |
224.2 g/mol | |
Source | PubChem | |
URL | https://pubchem.ncbi.nlm.nih.gov | |
Description | Data deposited in or computed by PubChem | |
Physical Description |
Gray powder; [Alfa Aesar MSDS] | |
Record name | Zinc nitride | |
Source | Haz-Map, Information on Hazardous Chemicals and Occupational Diseases | |
URL | https://haz-map.com/Agents/9710 | |
Description | Haz-Map® is an occupational health database designed for health and safety professionals and for consumers seeking information about the adverse effects of workplace exposures to chemical and biological agents. | |
Explanation | Copyright (c) 2022 Haz-Map(R). All rights reserved. Unless otherwise indicated, all materials from Haz-Map are copyrighted by Haz-Map(R). No part of these materials, either text or image may be used for any purpose other than for personal use. Therefore, reproduction, modification, storage in a retrieval system or retransmission, in any form or by any means, electronic, mechanical or otherwise, for reasons other than personal use, is strictly prohibited without prior written permission. | |
CAS No. |
1313-49-1 | |
Record name | Zinc nitride (Zn3N2) | |
Source | EPA Chemicals under the TSCA | |
URL | https://www.epa.gov/chemicals-under-tsca | |
Description | EPA Chemicals under the Toxic Substances Control Act (TSCA) collection contains information on chemicals and their regulations under TSCA, including non-confidential content from the TSCA Chemical Substance Inventory and Chemical Data Reporting. | |
Record name | Trizinc dinitride | |
Source | European Chemicals Agency (ECHA) | |
URL | https://echa.europa.eu/substance-information/-/substanceinfo/100.013.826 | |
Description | The European Chemicals Agency (ECHA) is an agency of the European Union which is the driving force among regulatory authorities in implementing the EU's groundbreaking chemicals legislation for the benefit of human health and the environment as well as for innovation and competitiveness. | |
Explanation | Use of the information, documents and data from the ECHA website is subject to the terms and conditions of this Legal Notice, and subject to other binding limitations provided for under applicable law, the information, documents and data made available on the ECHA website may be reproduced, distributed and/or used, totally or in part, for non-commercial purposes provided that ECHA is acknowledged as the source: "Source: European Chemicals Agency, http://echa.europa.eu/". Such acknowledgement must be included in each copy of the material. ECHA permits and encourages organisations and individuals to create links to the ECHA website under the following cumulative conditions: Links can only be made to webpages that provide a link to the Legal Notice page. | |
Disclaimer and Information on In-Vitro Research Products
Please be aware that all articles and product information presented on BenchChem are intended solely for informational purposes. The products available for purchase on BenchChem are specifically designed for in-vitro studies, which are conducted outside of living organisms. In-vitro studies, derived from the Latin term "in glass," involve experiments performed in controlled laboratory settings using cells or tissues. It is important to note that these products are not categorized as medicines or drugs, and they have not received approval from the FDA for the prevention, treatment, or cure of any medical condition, ailment, or disease. We must emphasize that any form of bodily introduction of these products into humans or animals is strictly prohibited by law. It is essential to adhere to these guidelines to ensure compliance with legal and ethical standards in research and experimentation.