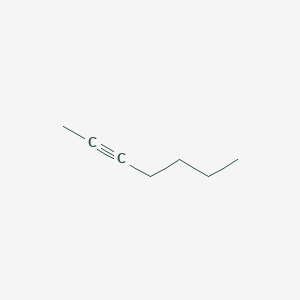
2-Heptyne
Overview
Description
2-Heptyne (CAS 1119-65-9) is a terminal alkyne with the molecular formula C₇H₁₂ and a linear structure (CCCCC#CC) . Key properties include:
- Molecular weight: 96.17 g/mol
- Boiling point: 111.9°C at 760 mmHg
- Density: 0.754 g/cm³
- Thermodynamic data:
It is used in laboratory synthesis and industrial applications, such as alkyne metathesis, where it forms as a byproduct .
Preparation Methods
Synthetic Routes and Reaction Conditions: 2-Heptyne can be synthesized through various methods, including the dehydrohalogenation of dihalides. One common approach involves the double elimination reaction of a vicinal dihalide or a geminal dihalide using a strong base such as sodium amide in liquid ammonia. This process results in the formation of the triple bond characteristic of alkynes .
Industrial Production Methods: In industrial settings, this compound can be produced by the catalytic dehydrogenation of heptane or by the partial hydrogenation of heptadiene. These methods typically involve the use of metal catalysts such as palladium or platinum to facilitate the removal of hydrogen atoms and the formation of the triple bond.
Chemical Reactions Analysis
Types of Reactions: 2-Heptyne undergoes a variety of chemical reactions, including:
Addition Reactions: The triple bond in this compound is highly reactive and can participate in addition reactions with halogens, hydrogen halides, and other electrophiles.
Oxidation Reactions: this compound can be oxidized to form diketones or carboxylic acids using oxidizing agents such as potassium permanganate or ozone.
Reduction Reactions: The compound can be reduced to form alkenes or alkanes using hydrogen gas in the presence of metal catalysts like palladium on carbon.
Common Reagents and Conditions:
Halogenation: Chlorine or bromine in the presence of a solvent like carbon tetrachloride.
Hydrogenation: Hydrogen gas with palladium or platinum catalysts.
Oxidation: Potassium permanganate in an aqueous medium.
Major Products Formed:
Dihalides: From halogenation reactions.
Alkanes and Alkenes: From reduction reactions.
Diketones and Carboxylic Acids: From oxidation reactions.
Scientific Research Applications
2-Heptyne has several applications in scientific research, including:
Organic Synthesis: It serves as a building block for the synthesis of more complex organic molecules.
Material Science: Used in the production of polymers and other advanced materials.
Pharmaceuticals: Acts as an intermediate in the synthesis of various drugs and bioactive compounds.
Catalysis Research: Studied for its reactivity and potential use in catalytic processes
Mechanism of Action
The mechanism of action of 2-Heptyne in chemical reactions involves the interaction of its triple bond with various reagents. The triple bond acts as a nucleophile, attacking electrophilic species and forming addition products. In reduction reactions, the triple bond is hydrogenated to form alkenes or alkanes, while in oxidation reactions, it is cleaved to form diketones or carboxylic acids .
Comparison with Similar Compounds
Structural Isomers
1-Heptyne
- Structure : Terminal alkyne (HC≡C-C₅H₁₁).
- Properties: Higher acidity (terminal H) compared to internal isomers. Reacts readily in Sonogashira coupling. internal triple bond positions .
3-Heptyne (CAS 2586-89-2)
- Structure : Internal alkyne (CH₂CH₂C≡C-C₃H₇).
- Properties :
Branched Isomers
5-Methyl-2-heptyne (CAS not provided)
- Structure : Branched internal alkyne (CH₂C≡C-CH(CH₃)-C₃H₇).
- Molecular formula : C₈H₁₄.
- Molecular weight : 110.20 g/mol .
- Key differences :
Other Alkynes of Similar Chain Length
2-Hexyne
- Structure : Shorter chain (C₆H₁₀).
- Comparison : Lower molecular weight (82.15 g/mol) and boiling point (~84°C). Reactivity differences arise from chain length, e.g., in polymerization rates .
Data Tables
Table 1: Physical and Thermodynamic Properties
Table 2: Structural and Reactivity Comparison
Research Findings
- Catalytic Reactivity: this compound forms during metathesis of 4-nonyne, suggesting its role as an intermediate in complex alkyne transformations .
- Thermodynamic Stability : The lower hydrogenation enthalpy of 3-heptyne compared to this compound reflects reduced stability due to internal triple bond positioning .
- Industrial Applications : Branched isomers like 5-methyl-2-heptyne are valued for tailored polymer synthesis, where steric effects modulate reaction pathways .
Biological Activity
2-Heptyne is an alkyne with the molecular formula . It is a linear chain hydrocarbon featuring a triple bond between the second and third carbon atoms. This compound has garnered interest in various fields, including medicinal chemistry, due to its potential biological activities. This article explores the biological activity of this compound, including its pharmacological properties, toxicity, and potential therapeutic applications.
Cytotoxicity and Antimicrobial Properties
Recent studies have indicated that this compound exhibits significant cytotoxic activity against various cell lines. In particular, it has been tested against cancer cell lines and shown to induce apoptosis in a concentration-dependent manner. The following table summarizes findings related to its cytotoxic effects:
The mechanism by which this compound exerts its cytotoxic effects appears to involve the generation of reactive oxygen species (ROS), leading to oxidative stress and subsequent apoptosis in cancer cells. Studies utilizing flow cytometry have demonstrated increased levels of annexin V staining in treated cells, indicating early apoptotic events.
Antimicrobial Activity
In addition to its cytotoxic properties, this compound has been evaluated for its antimicrobial activity. It has shown effectiveness against several bacterial strains, including:
- Staphylococcus aureus : Minimum Inhibitory Concentration (MIC) of 32 µg/mL.
- Escherichia coli : MIC of 64 µg/mL.
These findings suggest that this compound could serve as a potential lead compound for developing new antimicrobial agents.
Toxicity Studies
Toxicological assessments of this compound indicate that while it possesses significant biological activity, it also exhibits toxicity at higher concentrations. In animal studies, doses above 100 mg/kg resulted in noticeable adverse effects, including neurotoxicity and hepatotoxicity. The following table summarizes key findings from toxicity studies:
Endpoint | Observed Effect | Dose (mg/kg) |
---|---|---|
Neurotoxicity | Motor impairment | >100 |
Hepatotoxicity | Liver enzyme elevation | >100 |
General toxicity | Lethargy | >150 |
Case Studies
Several case studies have explored the application of this compound in therapeutic settings. One notable study involved the use of this compound derivatives in treating bacterial infections resistant to conventional antibiotics. The derivatives demonstrated enhanced efficacy compared to their parent compounds, highlighting the potential for structural modifications to improve biological activity.
Q & A
Basic Research Questions
Q. What are the recommended methods for synthesizing 2-Heptyne in a laboratory setting?
- This compound (CAS 1119-65-9) is typically synthesized via alkyne alkylation or dehydrohalogenation of vicinal dihalides. A common approach involves coupling terminal alkynes with alkyl halides using a strong base (e.g., NaNH₂ in liquid NH₃). Experimental protocols should emphasize inert atmosphere conditions to prevent oxidation or side reactions. Characterization via GC-MS and NMR is critical to confirm purity and structure . Safety protocols must address its flammability (flash point: 11°C) and inhalation hazards .
Q. How can the purity of this compound be assessed using spectroscopic and chromatographic methods?
- Gas Chromatography (GC): Quantify purity by comparing retention times with standards and integrating peak areas.
- Infrared Spectroscopy (IR): Confirm the C≡C stretch at ~2100–2260 cm⁻¹ and absence of O-H or C=O stretches from contaminants .
- ¹H NMR: Verify the absence of proton signals near δ 1.5–2.5 ppm (indicative of alkene impurities) and match splitting patterns to the expected structure (e.g., terminal methyl groups at δ 0.9–1.1 ppm) .
Q. What safety protocols are critical when handling this compound in experimental workflows?
- PPE: Use nitrile gloves (tested per EN 374), flame-resistant lab coats, and safety goggles. Respiratory protection (e.g., NIOSH-approved masks) is mandatory if ventilation is inadequate .
- Storage: Keep in airtight containers under nitrogen, away from oxidizers and ignition sources. Conduct risk assessments for spill containment (use dry sand or inert absorbents) and emergency ventilation .
Advanced Research Questions
Q. How do computational models predict the reactivity of this compound in hydrogenation reactions compared to experimental data?
- Quantum chemical calculations (e.g., DFT) can model hydrogenation pathways, predicting activation energies and regioselectivity. Experimental validation involves measuring reaction kinetics (e.g., using calorimetry) and comparing ΔrH° values. For this compound hydrogenation to heptane, Rogers et al. (1979) reported ΔrH° = -272.4 ± 1.3 kJ/mol in hexane, aligning with computational predictions of exothermicity . Discrepancies may arise from solvent effects or catalyst choice, requiring iterative model refinement .
Q. What strategies resolve contradictions in reported thermodynamic properties of this compound (e.g., boiling point variations)?
- Data Triangulation: Compare values from multiple sources (e.g., NIST lists Tboil = 350 ± 200 K, while Aladdin reports 110°C). Assess experimental conditions (pressure, purity) and methodologies (distillation vs. dynamic vapor pressure measurements) .
- Error Analysis: Statistical tools (e.g., Grubbs’ test) can identify outliers. For example, the wide boiling point range suggests inconsistent purity controls; replicate studies under standardized conditions are advised .
Q. How can reaction kinetics of this compound with electrophilic reagents be systematically studied?
- Experimental Design:
- Variables: Catalyst type (e.g., Pd/C vs. Lindlar), solvent polarity, temperature.
- Controls: Monitor side products (e.g., over-reduction to alkanes) via GC or HPLC.
- Kinetic Profiling: Use in-situ IR or UV-Vis spectroscopy to track C≡C bond consumption.
Q. Data Contradiction Analysis
Q. How should researchers address discrepancies in the enthalpy of formation (ΔfH°) of this compound across studies?
- NIST reports ΔfH°gas = 84.8 ± 2.2 kJ/mol via calorimetry , while computational studies (e.g., QSPR) may yield divergent values. Cross-validate using:
- Benson Group Additivity: Estimate ΔfH° based on bond contributions and compare with experimental data.
- High-Level Ab Initio Methods: CCSD(T)/CBS calculations reduce systematic errors. Discrepancies >5 kJ/mol warrant re-evaluation of experimental calibration (e.g., impurity corrections) .
Q. Methodological Guidance
Q. What protocols ensure reproducibility in catalytic hydrogenation studies of this compound?
- Catalyst Activation: Pre-reduce Pd/C or Ni catalysts under H₂ flow (1–2 atm, 100°C) to remove surface oxides.
- Reaction Monitoring: Use inline FTIR to detect intermediate alkenes and optimize H₂ pressure (prevents over-reduction).
- Reporting Standards: Document solvent degassing methods, catalyst loading (wt%), and stirring rates to align with Beilstein Journal guidelines .
Q. Tables of Key Data
Properties
IUPAC Name |
hept-2-yne | |
---|---|---|
Source | PubChem | |
URL | https://pubchem.ncbi.nlm.nih.gov | |
Description | Data deposited in or computed by PubChem | |
InChI |
InChI=1S/C7H12/c1-3-5-7-6-4-2/h3,5,7H2,1-2H3 | |
Source | PubChem | |
URL | https://pubchem.ncbi.nlm.nih.gov | |
Description | Data deposited in or computed by PubChem | |
InChI Key |
AMSFEMSYKQQCHL-UHFFFAOYSA-N | |
Source | PubChem | |
URL | https://pubchem.ncbi.nlm.nih.gov | |
Description | Data deposited in or computed by PubChem | |
Canonical SMILES |
CCCCC#CC | |
Source | PubChem | |
URL | https://pubchem.ncbi.nlm.nih.gov | |
Description | Data deposited in or computed by PubChem | |
Molecular Formula |
C7H12 | |
Source | PubChem | |
URL | https://pubchem.ncbi.nlm.nih.gov | |
Description | Data deposited in or computed by PubChem | |
DSSTOX Substance ID |
DTXSID90149770 | |
Record name | Hept-2-yne | |
Source | EPA DSSTox | |
URL | https://comptox.epa.gov/dashboard/DTXSID90149770 | |
Description | DSSTox provides a high quality public chemistry resource for supporting improved predictive toxicology. | |
Molecular Weight |
96.17 g/mol | |
Source | PubChem | |
URL | https://pubchem.ncbi.nlm.nih.gov | |
Description | Data deposited in or computed by PubChem | |
CAS No. |
1119-65-9 | |
Record name | 2-Heptyne | |
Source | CAS Common Chemistry | |
URL | https://commonchemistry.cas.org/detail?cas_rn=1119-65-9 | |
Description | CAS Common Chemistry is an open community resource for accessing chemical information. Nearly 500,000 chemical substances from CAS REGISTRY cover areas of community interest, including common and frequently regulated chemicals, and those relevant to high school and undergraduate chemistry classes. This chemical information, curated by our expert scientists, is provided in alignment with our mission as a division of the American Chemical Society. | |
Explanation | The data from CAS Common Chemistry is provided under a CC-BY-NC 4.0 license, unless otherwise stated. | |
Record name | Hept-2-yne | |
Source | ChemIDplus | |
URL | https://pubchem.ncbi.nlm.nih.gov/substance/?source=chemidplus&sourceid=0001119659 | |
Description | ChemIDplus is a free, web search system that provides access to the structure and nomenclature authority files used for the identification of chemical substances cited in National Library of Medicine (NLM) databases, including the TOXNET system. | |
Record name | 2-HEPTYNE | |
Source | DTP/NCI | |
URL | https://dtp.cancer.gov/dtpstandard/servlet/dwindex?searchtype=NSC&outputformat=html&searchlist=63874 | |
Description | The NCI Development Therapeutics Program (DTP) provides services and resources to the academic and private-sector research communities worldwide to facilitate the discovery and development of new cancer therapeutic agents. | |
Explanation | Unless otherwise indicated, all text within NCI products is free of copyright and may be reused without our permission. Credit the National Cancer Institute as the source. | |
Record name | Hept-2-yne | |
Source | EPA DSSTox | |
URL | https://comptox.epa.gov/dashboard/DTXSID90149770 | |
Description | DSSTox provides a high quality public chemistry resource for supporting improved predictive toxicology. | |
Record name | Hept-2-yne | |
Source | European Chemicals Agency (ECHA) | |
URL | https://echa.europa.eu/substance-information/-/substanceinfo/100.012.987 | |
Description | The European Chemicals Agency (ECHA) is an agency of the European Union which is the driving force among regulatory authorities in implementing the EU's groundbreaking chemicals legislation for the benefit of human health and the environment as well as for innovation and competitiveness. | |
Explanation | Use of the information, documents and data from the ECHA website is subject to the terms and conditions of this Legal Notice, and subject to other binding limitations provided for under applicable law, the information, documents and data made available on the ECHA website may be reproduced, distributed and/or used, totally or in part, for non-commercial purposes provided that ECHA is acknowledged as the source: "Source: European Chemicals Agency, http://echa.europa.eu/". Such acknowledgement must be included in each copy of the material. ECHA permits and encourages organisations and individuals to create links to the ECHA website under the following cumulative conditions: Links can only be made to webpages that provide a link to the Legal Notice page. | |
Retrosynthesis Analysis
AI-Powered Synthesis Planning: Our tool employs the Template_relevance Pistachio, Template_relevance Bkms_metabolic, Template_relevance Pistachio_ringbreaker, Template_relevance Reaxys, Template_relevance Reaxys_biocatalysis model, leveraging a vast database of chemical reactions to predict feasible synthetic routes.
One-Step Synthesis Focus: Specifically designed for one-step synthesis, it provides concise and direct routes for your target compounds, streamlining the synthesis process.
Accurate Predictions: Utilizing the extensive PISTACHIO, BKMS_METABOLIC, PISTACHIO_RINGBREAKER, REAXYS, REAXYS_BIOCATALYSIS database, our tool offers high-accuracy predictions, reflecting the latest in chemical research and data.
Strategy Settings
Precursor scoring | Relevance Heuristic |
---|---|
Min. plausibility | 0.01 |
Model | Template_relevance |
Template Set | Pistachio/Bkms_metabolic/Pistachio_ringbreaker/Reaxys/Reaxys_biocatalysis |
Top-N result to add to graph | 6 |
Feasible Synthetic Routes
Disclaimer and Information on In-Vitro Research Products
Please be aware that all articles and product information presented on BenchChem are intended solely for informational purposes. The products available for purchase on BenchChem are specifically designed for in-vitro studies, which are conducted outside of living organisms. In-vitro studies, derived from the Latin term "in glass," involve experiments performed in controlled laboratory settings using cells or tissues. It is important to note that these products are not categorized as medicines or drugs, and they have not received approval from the FDA for the prevention, treatment, or cure of any medical condition, ailment, or disease. We must emphasize that any form of bodily introduction of these products into humans or animals is strictly prohibited by law. It is essential to adhere to these guidelines to ensure compliance with legal and ethical standards in research and experimentation.