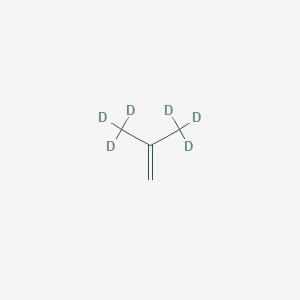
3,3,3-Trideuterio-2-(trideuteriomethyl)prop-1-ene
Overview
Description
3,3,3-Trideuterio-2-(trideuteriomethyl)prop-1-ene is a deuterated derivative of 2-methylpropene, commonly known as isobutylene. This compound is characterized by the replacement of hydrogen atoms with deuterium, a stable isotope of hydrogen. The molecular formula of this compound is C4H8, and it has a molecular weight of 62.14 g/mol.
Preparation Methods
Synthetic Routes and Reaction Conditions
The synthesis of 3,3,3-Trideuterio-2-(trideuteriomethyl)prop-1-ene typically involves the deuteration of 2-methylpropene. This process can be achieved through catalytic hydrogenation using deuterium gas (D2) in the presence of a suitable catalyst such as palladium on carbon (Pd/C). The reaction is carried out under controlled conditions of temperature and pressure to ensure complete deuteration.
Industrial Production Methods
In an industrial setting, the production of this compound follows a similar approach but on a larger scale. The process involves the use of high-pressure reactors and continuous flow systems to optimize the yield and purity of the compound. The deuterium gas used in the process is often sourced from heavy water (D2O), which is electrolyzed to produce deuterium gas.
Chemical Reactions Analysis
Types of Reactions
3,3,3-Trideuterio-2-(trideuteriomethyl)prop-1-ene undergoes various chemical reactions, including:
Oxidation: The compound can be oxidized to form corresponding alcohols, aldehydes, or carboxylic acids.
Reduction: Reduction reactions can convert the compound into saturated hydrocarbons.
Substitution: The compound can undergo substitution reactions where deuterium atoms are replaced by other functional groups.
Common Reagents and Conditions
Oxidation: Common oxidizing agents include potassium permanganate (KMnO4) and chromium trioxide (CrO3).
Reduction: Catalytic hydrogenation using hydrogen gas (H2) and a catalyst such as palladium on carbon (Pd/C).
Substitution: Reagents such as halogens (e.g., chlorine, bromine) and nucleophiles (e.g., hydroxide ions, alkoxide ions) are used.
Major Products Formed
Oxidation: Formation of deuterated alcohols, aldehydes, or carboxylic acids.
Reduction: Formation of deuterated saturated hydrocarbons.
Substitution: Formation of deuterated halides or other substituted derivatives.
Scientific Research Applications
3,3,3-Trideuterio-2-(trideuteriomethyl)prop-1-ene has several scientific research applications, including:
Chemistry: Used as a tracer in reaction mechanisms to study the kinetic isotope effect.
Biology: Employed in metabolic studies to trace the incorporation of deuterium into biological molecules.
Medicine: Utilized in the development of deuterated drugs, which may exhibit improved pharmacokinetic properties.
Industry: Applied in the production of synthetic rubber, plastics, and fuel additives.
Mechanism of Action
The mechanism of action of 3,3,3-Trideuterio-2-(trideuteriomethyl)prop-1-ene involves the incorporation of deuterium atoms into chemical reactions. Deuterium, being heavier than hydrogen, affects the reaction kinetics and can slow down certain reaction pathways. This property is exploited in studies of reaction mechanisms and in the development of deuterated drugs, where the presence of deuterium can enhance the stability and efficacy of the drug.
Comparison with Similar Compounds
Similar Compounds
2-Methylpropene (Isobutylene): The non-deuterated form of 3,3,3-Trideuterio-2-(trideuteriomethyl)prop-1-ene.
3,3,3-Trideuterio-2-methylprop-1-ene: Another deuterated derivative with a similar structure.
Uniqueness
This compound is unique due to the presence of multiple deuterium atoms, which makes it particularly useful in studies involving the kinetic isotope effect. The compound’s deuterium content also enhances its stability and resistance to metabolic degradation, making it valuable in pharmaceutical research and development.
Properties
IUPAC Name |
3,3,3-trideuterio-2-(trideuteriomethyl)prop-1-ene | |
---|---|---|
Source | PubChem | |
URL | https://pubchem.ncbi.nlm.nih.gov | |
Description | Data deposited in or computed by PubChem | |
InChI |
InChI=1S/C4H8/c1-4(2)3/h1H2,2-3H3/i2D3,3D3 | |
Source | PubChem | |
URL | https://pubchem.ncbi.nlm.nih.gov | |
Description | Data deposited in or computed by PubChem | |
InChI Key |
VQTUBCCKSQIDNK-XERRXZQWSA-N | |
Source | PubChem | |
URL | https://pubchem.ncbi.nlm.nih.gov | |
Description | Data deposited in or computed by PubChem | |
Canonical SMILES |
CC(=C)C | |
Source | PubChem | |
URL | https://pubchem.ncbi.nlm.nih.gov | |
Description | Data deposited in or computed by PubChem | |
Isomeric SMILES |
[2H]C([2H])([2H])C(=C)C([2H])([2H])[2H] | |
Source | PubChem | |
URL | https://pubchem.ncbi.nlm.nih.gov | |
Description | Data deposited in or computed by PubChem | |
Molecular Formula |
C4H8 | |
Source | PubChem | |
URL | https://pubchem.ncbi.nlm.nih.gov | |
Description | Data deposited in or computed by PubChem | |
Molecular Weight |
62.14 g/mol | |
Source | PubChem | |
URL | https://pubchem.ncbi.nlm.nih.gov | |
Description | Data deposited in or computed by PubChem | |
Synthesis routes and methods I
Procedure details
Synthesis routes and methods II
Procedure details
Synthesis routes and methods III
Procedure details
Synthesis routes and methods IV
Procedure details
Synthesis routes and methods V
Procedure details
Retrosynthesis Analysis
AI-Powered Synthesis Planning: Our tool employs the Template_relevance Pistachio, Template_relevance Bkms_metabolic, Template_relevance Pistachio_ringbreaker, Template_relevance Reaxys, Template_relevance Reaxys_biocatalysis model, leveraging a vast database of chemical reactions to predict feasible synthetic routes.
One-Step Synthesis Focus: Specifically designed for one-step synthesis, it provides concise and direct routes for your target compounds, streamlining the synthesis process.
Accurate Predictions: Utilizing the extensive PISTACHIO, BKMS_METABOLIC, PISTACHIO_RINGBREAKER, REAXYS, REAXYS_BIOCATALYSIS database, our tool offers high-accuracy predictions, reflecting the latest in chemical research and data.
Strategy Settings
Precursor scoring | Relevance Heuristic |
---|---|
Min. plausibility | 0.01 |
Model | Template_relevance |
Template Set | Pistachio/Bkms_metabolic/Pistachio_ringbreaker/Reaxys/Reaxys_biocatalysis |
Top-N result to add to graph | 6 |
Feasible Synthetic Routes
Disclaimer and Information on In-Vitro Research Products
Please be aware that all articles and product information presented on BenchChem are intended solely for informational purposes. The products available for purchase on BenchChem are specifically designed for in-vitro studies, which are conducted outside of living organisms. In-vitro studies, derived from the Latin term "in glass," involve experiments performed in controlled laboratory settings using cells or tissues. It is important to note that these products are not categorized as medicines or drugs, and they have not received approval from the FDA for the prevention, treatment, or cure of any medical condition, ailment, or disease. We must emphasize that any form of bodily introduction of these products into humans or animals is strictly prohibited by law. It is essential to adhere to these guidelines to ensure compliance with legal and ethical standards in research and experimentation.