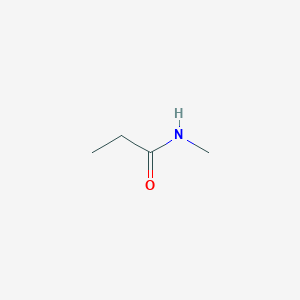
N-Methylpropionamide
Overview
Description
N-Methylpropionamide, also known as N-Methylpropionic acid amide, is an organic compound with the molecular formula C4H9NO. It is a clear, colorless liquid at room temperature and is known for its high dielectric constant. The compound is used in various scientific and industrial applications due to its unique chemical properties.
Mechanism of Action
Target of Action
N-Methylpropionamide, also known as N-Methylpropanamide, is an organic chemical compound with the molecular formula C4H9NO
Pharmacokinetics
Its physical properties such as boiling point (146 °c/90 mmhg) and density (0.931 g/mL at 25 °C) suggest that it may have certain bioavailability characteristics common to other small organic compounds.
Preparation Methods
Synthetic Routes and Reaction Conditions: N-Methylpropionamide can be synthesized through the condensation of anhydrous methylamine with propionic acid. The reaction is typically carried out with a 50% excess of propionic acid to ensure complete conversion. Rapid heating to 120-140°C with stirring helps in removing water either directly or as a ternary xylene azeotrope .
Industrial Production Methods: In industrial settings, this compound is often produced by the same condensation reaction but on a larger scale. The product is purified by distillation under reduced pressure and dried over calcium oxide to remove any residual water and unreacted propionic acid .
Chemical Reactions Analysis
Types of Reactions: N-Methylpropionamide undergoes various chemical reactions, including:
Oxidation: It can be oxidized to form N-methylpropionic acid.
Reduction: Reduction reactions can convert it to N-methylpropanamine.
Substitution: It can participate in nucleophilic substitution reactions, where the amide group is replaced by other functional groups.
Common Reagents and Conditions:
Oxidation: Common oxidizing agents include potassium permanganate and chromium trioxide.
Reduction: Reducing agents such as lithium aluminum hydride or sodium borohydride are used.
Substitution: Reagents like alkyl halides or acyl chlorides can be used under basic or acidic conditions.
Major Products:
Oxidation: N-Methylpropionic acid.
Reduction: N-Methylpropanamine.
Substitution: Various substituted amides depending on the reagents used.
Scientific Research Applications
N-Methylpropionamide has several applications in scientific research:
Chemistry: It is used as a solvent and reagent in organic synthesis.
Medicine: Research is ongoing to explore its potential therapeutic applications.
Industry: It is used in the production of various chemicals and as an electrolyte solvent.
Comparison with Similar Compounds
- N,N-Dimethylpropionamide
- N-Methylacetamide
- N-Ethylpropionamide
Comparison: N-Methylpropionamide is unique due to its specific molecular structure, which imparts distinct chemical properties such as a high dielectric constant and specific reactivity patterns. Compared to N,N-Dimethylpropionamide, it has a simpler structure, making it easier to handle and use in various applications .
Properties
IUPAC Name |
N-methylpropanamide | |
---|---|---|
Source | PubChem | |
URL | https://pubchem.ncbi.nlm.nih.gov | |
Description | Data deposited in or computed by PubChem | |
InChI |
InChI=1S/C4H9NO/c1-3-4(6)5-2/h3H2,1-2H3,(H,5,6) | |
Source | PubChem | |
URL | https://pubchem.ncbi.nlm.nih.gov | |
Description | Data deposited in or computed by PubChem | |
InChI Key |
QJQAMHYHNCADNR-UHFFFAOYSA-N | |
Source | PubChem | |
URL | https://pubchem.ncbi.nlm.nih.gov | |
Description | Data deposited in or computed by PubChem | |
Canonical SMILES |
CCC(=O)NC | |
Source | PubChem | |
URL | https://pubchem.ncbi.nlm.nih.gov | |
Description | Data deposited in or computed by PubChem | |
Molecular Formula |
C4H9NO | |
Source | PubChem | |
URL | https://pubchem.ncbi.nlm.nih.gov | |
Description | Data deposited in or computed by PubChem | |
DSSTOX Substance ID |
DTXSID0061583 | |
Record name | Propanamide, N-methyl- | |
Source | EPA DSSTox | |
URL | https://comptox.epa.gov/dashboard/DTXSID0061583 | |
Description | DSSTox provides a high quality public chemistry resource for supporting improved predictive toxicology. | |
Molecular Weight |
87.12 g/mol | |
Source | PubChem | |
URL | https://pubchem.ncbi.nlm.nih.gov | |
Description | Data deposited in or computed by PubChem | |
CAS No. |
1187-58-2 | |
Record name | N-Methylpropionamide | |
Source | CAS Common Chemistry | |
URL | https://commonchemistry.cas.org/detail?cas_rn=1187-58-2 | |
Description | CAS Common Chemistry is an open community resource for accessing chemical information. Nearly 500,000 chemical substances from CAS REGISTRY cover areas of community interest, including common and frequently regulated chemicals, and those relevant to high school and undergraduate chemistry classes. This chemical information, curated by our expert scientists, is provided in alignment with our mission as a division of the American Chemical Society. | |
Explanation | The data from CAS Common Chemistry is provided under a CC-BY-NC 4.0 license, unless otherwise stated. | |
Record name | N-Methylpropanamide | |
Source | ChemIDplus | |
URL | https://pubchem.ncbi.nlm.nih.gov/substance/?source=chemidplus&sourceid=0001187582 | |
Description | ChemIDplus is a free, web search system that provides access to the structure and nomenclature authority files used for the identification of chemical substances cited in National Library of Medicine (NLM) databases, including the TOXNET system. | |
Record name | N-Methylpropionamide | |
Source | DTP/NCI | |
URL | https://dtp.cancer.gov/dtpstandard/servlet/dwindex?searchtype=NSC&outputformat=html&searchlist=54114 | |
Description | The NCI Development Therapeutics Program (DTP) provides services and resources to the academic and private-sector research communities worldwide to facilitate the discovery and development of new cancer therapeutic agents. | |
Explanation | Unless otherwise indicated, all text within NCI products is free of copyright and may be reused without our permission. Credit the National Cancer Institute as the source. | |
Record name | Propanamide, N-methyl- | |
Source | EPA Chemicals under the TSCA | |
URL | https://www.epa.gov/chemicals-under-tsca | |
Description | EPA Chemicals under the Toxic Substances Control Act (TSCA) collection contains information on chemicals and their regulations under TSCA, including non-confidential content from the TSCA Chemical Substance Inventory and Chemical Data Reporting. | |
Record name | Propanamide, N-methyl- | |
Source | EPA DSSTox | |
URL | https://comptox.epa.gov/dashboard/DTXSID0061583 | |
Description | DSSTox provides a high quality public chemistry resource for supporting improved predictive toxicology. | |
Record name | N-methylpropionamide | |
Source | European Chemicals Agency (ECHA) | |
URL | https://echa.europa.eu/substance-information/-/substanceinfo/100.013.363 | |
Description | The European Chemicals Agency (ECHA) is an agency of the European Union which is the driving force among regulatory authorities in implementing the EU's groundbreaking chemicals legislation for the benefit of human health and the environment as well as for innovation and competitiveness. | |
Explanation | Use of the information, documents and data from the ECHA website is subject to the terms and conditions of this Legal Notice, and subject to other binding limitations provided for under applicable law, the information, documents and data made available on the ECHA website may be reproduced, distributed and/or used, totally or in part, for non-commercial purposes provided that ECHA is acknowledged as the source: "Source: European Chemicals Agency, http://echa.europa.eu/". Such acknowledgement must be included in each copy of the material. ECHA permits and encourages organisations and individuals to create links to the ECHA website under the following cumulative conditions: Links can only be made to webpages that provide a link to the Legal Notice page. | |
Record name | N-METHYLPROPANAMIDE | |
Source | FDA Global Substance Registration System (GSRS) | |
URL | https://gsrs.ncats.nih.gov/ginas/app/beta/substances/2TSZ8HW6DQ | |
Description | The FDA Global Substance Registration System (GSRS) enables the efficient and accurate exchange of information on what substances are in regulated products. Instead of relying on names, which vary across regulatory domains, countries, and regions, the GSRS knowledge base makes it possible for substances to be defined by standardized, scientific descriptions. | |
Explanation | Unless otherwise noted, the contents of the FDA website (www.fda.gov), both text and graphics, are not copyrighted. They are in the public domain and may be republished, reprinted and otherwise used freely by anyone without the need to obtain permission from FDA. Credit to the U.S. Food and Drug Administration as the source is appreciated but not required. | |
Synthesis routes and methods
Procedure details
Retrosynthesis Analysis
AI-Powered Synthesis Planning: Our tool employs the Template_relevance Pistachio, Template_relevance Bkms_metabolic, Template_relevance Pistachio_ringbreaker, Template_relevance Reaxys, Template_relevance Reaxys_biocatalysis model, leveraging a vast database of chemical reactions to predict feasible synthetic routes.
One-Step Synthesis Focus: Specifically designed for one-step synthesis, it provides concise and direct routes for your target compounds, streamlining the synthesis process.
Accurate Predictions: Utilizing the extensive PISTACHIO, BKMS_METABOLIC, PISTACHIO_RINGBREAKER, REAXYS, REAXYS_BIOCATALYSIS database, our tool offers high-accuracy predictions, reflecting the latest in chemical research and data.
Strategy Settings
Precursor scoring | Relevance Heuristic |
---|---|
Min. plausibility | 0.01 |
Model | Template_relevance |
Template Set | Pistachio/Bkms_metabolic/Pistachio_ringbreaker/Reaxys/Reaxys_biocatalysis |
Top-N result to add to graph | 6 |
Feasible Synthetic Routes
Q1: What is the molecular formula and weight of N-Methylpropionamide?
A1: this compound has the molecular formula C4H9NO and a molecular weight of 87.12 g/mol.
Q2: What spectroscopic techniques have been used to characterize this compound?
A2: Several spectroscopic methods have been employed to study this compound, including:
- NMR Spectroscopy: Used to analyze the structure and dynamics of this compound in solution, particularly focusing on hydrogen bonding interactions and molecular motions. [, , , , , ]
- Infrared (IR) Spectroscopy: Employed to investigate the characteristic vibrational modes of this compound, particularly the N–H and C=O stretching vibrations that provide insights into hydrogen bonding and molecular conformation. [, , ]
- Raman Spectroscopy: Utilized to study the vibrational modes of this compound, including preresonance and resonance Raman spectroscopy to enhance specific vibrational bands and provide information about molecular structure and interactions. [, , ]
- UV-Vis Spectroscopy: Used in studies involving the photodegradation of herbicides like metamifop, where this compound derivatives are formed as degradation products. UV-Vis spectroscopy aids in monitoring the degradation process and identifying the products. []
Q3: What is known about the dielectric properties of this compound?
A3: this compound exhibits a high dielectric constant, making it suitable as a solvent for electrolytic solutions. [, , ] The dielectric properties are influenced by temperature and the presence of other solvents like methanol. Studies suggest a homogeneous liquid structure with varying hydrogen bonding depending on the solvent composition. []
Q4: How does the molecular structure of this compound influence its interaction with water?
A4: this compound interacts with water through hydrogen bonding. The amide group (–CONH–) acts as both a hydrogen bond donor and acceptor. [] The hydrophobic ethyl group influences its solubility and interactions with hydrophobic molecules. []
Q5: What is known about the self-association of this compound in solution?
A5: this compound molecules can self-associate in solution through hydrogen bonding, forming dimers and larger aggregates. This self-association influences its physical properties, such as viscosity and dielectric relaxation. [, , ]
Q6: What are some applications of this compound as a solvent?
A6: Due to its high dielectric constant, this compound is used as a solvent for:
- Electrochemical studies: Investigating the behavior of electrolytes and conducting electrochemical reactions. [, , , ]
- Spectroscopic studies: Providing a polar environment for studying molecular interactions and dynamics using techniques like NMR and IR spectroscopy. [, , ]
Q7: How does this compound participate in chemical reactions?
A7: this compound can participate in several reactions, including:
- Hydrolysis: It can be hydrolyzed to propionic acid and methylamine, a reaction catalyzed by specific enzymes like theanine hydrolase found in tea leaves. []
- Hydrosilylation: The carbonyl group can undergo hydrosilylation reactions with hydrosilanes, catalyzed by transition metal complexes like triruthenium dodecacarbonyl. This reaction is promising for synthesizing new polymers and modifying material properties. [, ]
Q8: How do structural modifications to this compound affect its properties?
A8: Structural modifications, like changing the N-alkyl substituent or introducing electron-withdrawing groups, can significantly impact this compound's properties, including:
- Solubility: Increasing the alkyl chain length generally decreases water solubility. []
- Hydrogen bonding: The strength and nature of hydrogen bonding interactions. [, ]
- Chemical reactivity: For instance, introducing electron-withdrawing groups on the aromatic ring of N-phenylacetamide derivatives influences their reactivity in nucleophilic aromatic substitution reactions. [, ]
Q9: Does this compound exhibit any biological activity?
A9: While this compound itself hasn't been extensively studied for direct biological activity, some derivatives demonstrate significant effects:
- Protein Synthesis Inhibition: Compounds like 2-(4-methyl-2,6-dinitroanilino)-N-methylpropionamide (MDMP) are potent inhibitors of protein synthesis in plants and eukaryotic systems. MDMP specifically inhibits the initiation step of translation by interfering with the binding of mRNA to ribosomes. [, , , , , , ]
- Herbicidal activity: Metamifop, a herbicide containing the this compound moiety, effectively controls annual grass weeds in rice. []
Q10: How does MDMP, a derivative of this compound, affect mRNA stability?
A10: MDMP's inhibition of protein synthesis initiation, by reducing ribosome density on mRNA, can impact mRNA stability. Studies on Xenopus laevis vitellogenin mRNA revealed that maintaining a high ribosome density, not continuous protein synthesis, is crucial for estrogen-mediated mRNA stabilization. MDMP treatment led to vitellogenin mRNA degradation, suggesting a potential link between ribosome density and mRNA stability, independent of ongoing protein synthesis. []
Q11: What is known about the environmental fate of this compound and its derivatives?
A11: Research on the herbicide metamifop provides insights into the environmental fate of this compound derivatives. Studies show that metamifop undergoes photodegradation in water/acetonitrile solutions upon UV irradiation. [] The identified degradation products included N-(2-fluorophenyl)-2-hydroxy-N-methylpropionamide (HPFMA), suggesting further degradation pathways for these compounds in the environment. [, ]
Q12: Have computational methods been employed to study this compound?
A12: While the provided research doesn't explicitly detail computational studies on this compound itself, computational chemistry techniques like molecular dynamics (MD) simulations are applicable to study:
- Solvation dynamics: Simulating the behavior of this compound in various solvents and investigating the influence of hydrogen bonding on solvation properties. []
- Reaction mechanisms: Investigating the mechanisms of reactions involving this compound, such as hydrosilylation, to gain deeper insights into the reaction pathways and optimize reaction conditions. []
Q13: What are potential future research directions for this compound and its derivatives?
A13: Given the properties and applications highlighted, future research could explore:
- Developing new this compound-based polymers: Leveraging the hydrosilylation reaction to synthesize polymers with tailored properties for various applications. []
- Exploring the structure-activity relationship of MDMP analogs: Designing and synthesizing novel protein synthesis inhibitors with improved potency and selectivity for specific therapeutic targets. [, , , , , , ]
- Investigating the environmental fate and impact of this compound derivatives: Assessing the persistence, bioaccumulation, and potential ecotoxicological effects of these compounds in various environmental compartments. [, ]
Disclaimer and Information on In-Vitro Research Products
Please be aware that all articles and product information presented on BenchChem are intended solely for informational purposes. The products available for purchase on BenchChem are specifically designed for in-vitro studies, which are conducted outside of living organisms. In-vitro studies, derived from the Latin term "in glass," involve experiments performed in controlled laboratory settings using cells or tissues. It is important to note that these products are not categorized as medicines or drugs, and they have not received approval from the FDA for the prevention, treatment, or cure of any medical condition, ailment, or disease. We must emphasize that any form of bodily introduction of these products into humans or animals is strictly prohibited by law. It is essential to adhere to these guidelines to ensure compliance with legal and ethical standards in research and experimentation.