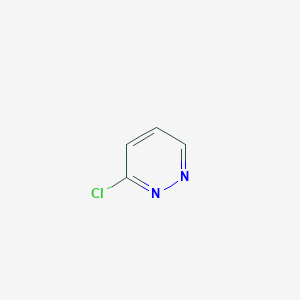
3-Chloropyridazine
Overview
Description
3-Chloropyridazine is an organic compound with the chemical formula C₄H₃ClN₂. It features a pyridazine ring, where a chlorine atom substitutes one of the hydrogen atoms at the third position. This compound is notable for its applications in various fields, including agriculture, pharmaceuticals, and chemical research.
Mechanism of Action
Target of Action
3-Chloropyridazine is a heterocyclic compound that contains two adjacent nitrogen atoms . It has been shown to have a wide range of pharmacological activities . .
Mode of Action
It’s known that pyridazine derivatives can inhibit calcium ion influx, which is required for the activation of platelet aggregation . This suggests that this compound might interact with its targets in a similar manner, leading to changes in cellular processes.
Biochemical Pathways
It’s known that pyridazine derivatives have a wide range of pharmacological activities, including antimicrobial, antidepressant, anti-hypertensive, anticancer, antiplatelet, antiulcer, herbicidal, antifeedant, and various other anticipated biological activities . This suggests that this compound might affect multiple biochemical pathways.
Pharmacokinetics
It’s known that the pharmaceutical industry has a continuing requirement for the synthesis of a diverse range of polysubstituted pyridazinone compounds bearing a varied range of ring substituents because many such compounds have been used as commercially drugs and agrochemicals .
Result of Action
It’s known that pyridazine derivatives can act as apoptotic inducers and parp-1 inhibitors . This suggests that this compound might have similar effects, leading to the induction of apoptosis and inhibition of PARP-1 in cells.
Biochemical Analysis
Biochemical Properties
3-Chloropyridazine, like other pyridazine derivatives, is known to interact with various enzymes, proteins, and other biomolecules
Cellular Effects
Pyridazine derivatives have been shown to exhibit a wide range of pharmacological activities such as antimicrobial, antidepressant, anti-hypertensive, anticancer, antiplatelet, antiulcer, herbicidal, antifeedant, and various other anticipated biological activities . These effects suggest that this compound may influence cell function, signaling pathways, gene expression, and cellular metabolism.
Molecular Mechanism
It has been suggested that pyridazine derivatives may exert their effects at the molecular level through binding interactions with biomolecules, enzyme inhibition or activation, and changes in gene expression .
Subcellular Localization
This could include any targeting signals or post-translational modifications that direct it to specific compartments or organelles .
Preparation Methods
Synthetic Routes and Reaction Conditions: 3-Chloropyridazine can be synthesized through several methods. One common approach involves the chlorination of pyridazine. This process typically uses chlorine gas or chlorinating agents such as phosphorus pentachloride (PCl₅) under controlled conditions to achieve the substitution at the third position .
Industrial Production Methods: In industrial settings, the production of this compound often involves large-scale chlorination reactions. The process is optimized for yield and purity, employing continuous flow reactors and advanced purification techniques to ensure the compound meets the required specifications for its various applications .
Chemical Reactions Analysis
Types of Reactions: 3-Chloropyridazine undergoes several types of chemical reactions, including:
Nucleophilic Substitution: The chlorine atom can be replaced by nucleophiles such as amines or thiols, forming various substituted pyridazines.
Reduction: The compound can be reduced to form 3-aminopyridazine using reducing agents like lithium aluminum hydride (LiAlH₄).
Electrophilic Substitution: Under certain conditions, electrophiles can attack the pyridazine ring, leading to further substitution reactions.
Common Reagents and Conditions:
Nucleophilic Substitution: Reagents like sodium amide (NaNH₂) or thiourea in polar solvents.
Reduction: Lithium aluminum hydride (LiAlH₄) in anhydrous ether.
Electrophilic Substitution: Electrophiles such as acyl chlorides or sulfonyl chlorides in the presence of a Lewis acid catalyst.
Major Products:
Nucleophilic Substitution: Substituted pyridazines with various functional groups.
Reduction: 3-Aminopyridazine.
Electrophilic Substitution: Various electrophile-substituted pyridazines.
Scientific Research Applications
3-Chloropyridazine has a wide range of applications in scientific research:
Chemistry: It serves as a building block for synthesizing more complex molecules, including heterocyclic compounds and pharmaceuticals.
Biology: Used in the study of enzyme inhibitors and receptor antagonists due to its ability to interact with biological macromolecules.
Medicine: Investigated for its potential use in developing new drugs, particularly in the treatment of cancer and infectious diseases.
Industry: Utilized as an intermediate in the production of agrochemicals, dyes, and other industrial chemicals.
Comparison with Similar Compounds
3-Chloropyridazine can be compared with other chlorinated pyridazines and related heterocyclic compounds:
Properties
IUPAC Name |
3-chloropyridazine | |
---|---|---|
Source | PubChem | |
URL | https://pubchem.ncbi.nlm.nih.gov | |
Description | Data deposited in or computed by PubChem | |
InChI |
InChI=1S/C4H3ClN2/c5-4-2-1-3-6-7-4/h1-3H | |
Source | PubChem | |
URL | https://pubchem.ncbi.nlm.nih.gov | |
Description | Data deposited in or computed by PubChem | |
InChI Key |
IBWYHNOFSKJKKY-UHFFFAOYSA-N | |
Source | PubChem | |
URL | https://pubchem.ncbi.nlm.nih.gov | |
Description | Data deposited in or computed by PubChem | |
Canonical SMILES |
C1=CC(=NN=C1)Cl | |
Source | PubChem | |
URL | https://pubchem.ncbi.nlm.nih.gov | |
Description | Data deposited in or computed by PubChem | |
Molecular Formula |
C4H3ClN2 | |
Source | PubChem | |
URL | https://pubchem.ncbi.nlm.nih.gov | |
Description | Data deposited in or computed by PubChem | |
DSSTOX Substance ID |
DTXSID60460767 | |
Record name | 3-Chloropyridazine | |
Source | EPA DSSTox | |
URL | https://comptox.epa.gov/dashboard/DTXSID60460767 | |
Description | DSSTox provides a high quality public chemistry resource for supporting improved predictive toxicology. | |
Molecular Weight |
114.53 g/mol | |
Source | PubChem | |
URL | https://pubchem.ncbi.nlm.nih.gov | |
Description | Data deposited in or computed by PubChem | |
CAS No. |
1120-95-2 | |
Record name | 3-Chloropyridazine | |
Source | EPA DSSTox | |
URL | https://comptox.epa.gov/dashboard/DTXSID60460767 | |
Description | DSSTox provides a high quality public chemistry resource for supporting improved predictive toxicology. | |
Record name | 3-chloropyridazine | |
Source | European Chemicals Agency (ECHA) | |
URL | https://echa.europa.eu/information-on-chemicals | |
Description | The European Chemicals Agency (ECHA) is an agency of the European Union which is the driving force among regulatory authorities in implementing the EU's groundbreaking chemicals legislation for the benefit of human health and the environment as well as for innovation and competitiveness. | |
Explanation | Use of the information, documents and data from the ECHA website is subject to the terms and conditions of this Legal Notice, and subject to other binding limitations provided for under applicable law, the information, documents and data made available on the ECHA website may be reproduced, distributed and/or used, totally or in part, for non-commercial purposes provided that ECHA is acknowledged as the source: "Source: European Chemicals Agency, http://echa.europa.eu/". Such acknowledgement must be included in each copy of the material. ECHA permits and encourages organisations and individuals to create links to the ECHA website under the following cumulative conditions: Links can only be made to webpages that provide a link to the Legal Notice page. | |
Retrosynthesis Analysis
AI-Powered Synthesis Planning: Our tool employs the Template_relevance Pistachio, Template_relevance Bkms_metabolic, Template_relevance Pistachio_ringbreaker, Template_relevance Reaxys, Template_relevance Reaxys_biocatalysis model, leveraging a vast database of chemical reactions to predict feasible synthetic routes.
One-Step Synthesis Focus: Specifically designed for one-step synthesis, it provides concise and direct routes for your target compounds, streamlining the synthesis process.
Accurate Predictions: Utilizing the extensive PISTACHIO, BKMS_METABOLIC, PISTACHIO_RINGBREAKER, REAXYS, REAXYS_BIOCATALYSIS database, our tool offers high-accuracy predictions, reflecting the latest in chemical research and data.
Strategy Settings
Precursor scoring | Relevance Heuristic |
---|---|
Min. plausibility | 0.01 |
Model | Template_relevance |
Template Set | Pistachio/Bkms_metabolic/Pistachio_ringbreaker/Reaxys/Reaxys_biocatalysis |
Top-N result to add to graph | 6 |
Feasible Synthetic Routes
Q1: What is the molecular formula and weight of 3-Chloropyridazine?
A1: The molecular formula of this compound is C4H3ClN2, and its molecular weight is 114.55 g/mol.
Q2: What spectroscopic data is available for characterizing this compound?
A2: Researchers commonly use infrared (IR), ultraviolet-visible (UV-Vis), and nuclear magnetic resonance (NMR) spectroscopy to characterize this compound. [, , , ] These techniques provide insights into the compound's functional groups, electronic transitions, and proton/carbon environments. [, , , ] For example, IR spectroscopy confirms the presence of specific functional groups like C-Cl and C=N bonds. [, , , ]
Q3: How does the chlorine atom in this compound influence its reactivity?
A3: The chlorine atom at the 3-position of the pyridazine ring acts as a good leaving group, making this compound susceptible to nucleophilic substitution reactions. [, , , , ] This reactivity allows for the introduction of diverse substituents at this position, leading to a wide range of pyridazine derivatives. [, , , , ]
Q4: What are some common reactions involving this compound as a starting material?
A4: this compound serves as a versatile starting material for synthesizing various heterocyclic compounds. Some common reactions include:
- Nucleophilic Substitution: Reaction with nucleophiles like amines, alkoxides, and thiols to replace the chlorine atom with the desired group. [, , , , ] This reaction is widely utilized to synthesize a variety of 3-substituted pyridazine derivatives. [, , , , ]
- Suzuki Coupling: Reaction with arylboronic acids in the presence of a palladium catalyst to form a carbon-carbon bond, allowing for the introduction of aryl groups at the 3-position. []
- Hydrazinolysis: Reaction with hydrazine to yield 3-hydrazinopyridazine, which can be further utilized to synthesize other heterocyclic systems. [, , , , , ]
Q5: Can you provide an example of a specific reaction where this compound is used to synthesize a biologically active compound?
A5: One example is the synthesis of 6-allylthio-3-aminopyridazine derivatives, potential anticancer agents. [] The synthesis involves reacting 3,6-dichloropyridazine with allylmercaptan to introduce the allylthio group, followed by amination at the 3-position using various heteroamines. []
Q6: What are the main applications of this compound and its derivatives?
A6: this compound derivatives exhibit diverse biological activities, making them attractive targets for pharmaceutical development. They have shown potential as:
- Antidepressants: Certain 3-aminopyridazine derivatives, structurally similar to minaprine, exhibit antidepressant activity by influencing cholinergic neurotransmission. [, ]
- Anticancer Agents: 6-Allylthio-3-aminopyridazine derivatives have shown promising anti-proliferative effects against MCF-7 human breast cancer cells. []
- Antimicrobial Agents: Various pyridazin-3(2H)-ones, 3-chloropyridazines, and pyridazin-3(2H)-thiones have demonstrated antibacterial activity against both Gram-positive and Gram-negative bacteria. []
- Interleukin-1β Converting Enzyme (ICE) Inhibitors: Some 3-chloropyridazines act as irreversible inactivators of ICE, potentially offering therapeutic benefits in inflammatory diseases and conditions involving dysregulated apoptosis. []
Q7: How do the substituents on the pyridazine ring affect the biological activity of this compound derivatives?
A7: The nature and position of substituents on the pyridazine ring significantly influence the biological activity of this compound derivatives. This structure-activity relationship (SAR) is crucial for designing compounds with improved potency, selectivity, and pharmacological properties. [, , , ]
Disclaimer and Information on In-Vitro Research Products
Please be aware that all articles and product information presented on BenchChem are intended solely for informational purposes. The products available for purchase on BenchChem are specifically designed for in-vitro studies, which are conducted outside of living organisms. In-vitro studies, derived from the Latin term "in glass," involve experiments performed in controlled laboratory settings using cells or tissues. It is important to note that these products are not categorized as medicines or drugs, and they have not received approval from the FDA for the prevention, treatment, or cure of any medical condition, ailment, or disease. We must emphasize that any form of bodily introduction of these products into humans or animals is strictly prohibited by law. It is essential to adhere to these guidelines to ensure compliance with legal and ethical standards in research and experimentation.