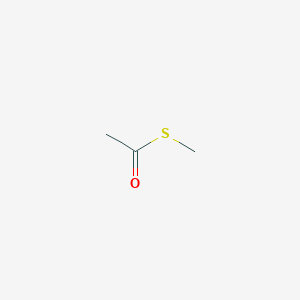
S-Methyl thioacetate
Overview
Description
S-Methyl thioacetate (CH₃C(O)SCH₃) is a sulfur-containing organic compound classified as a thioester. It is characterized by a carbonyl group adjacent to a thioether moiety, which confers unique chemical and biochemical reactivity. This compound is biosynthesized by microbial pathways, notably in Brevibacterium species via L-leucine catabolism, where it arises alongside branched-chain thioesters like S-methyl thioisovalerate and S-methyl thioisobutyrate . It is also synthesized abiotically through nickel-catalyzed reactions between acetylene (HC≡CH) and methyl mercaptan (CH₃SH) under hydrothermal conditions .
This compound is a key contributor to flavors and aromas in fermented foods (e.g., kimchi, cheese, and Chinese pickles) but can also impart off-flavors in seafood products like sea urchin gonads . Spectroscopic studies (infrared and Raman) confirm that its carbonyl group resembles ketones in reactivity, while the C(O)-S bond lacks double-bond character, distinguishing it from oxygen-based esters .
Preparation Methods
Enzymatic Synthesis via Microbial Pathways
Substrate-Specific Enzymatic Reactions
The enzymatic synthesis of MTA has been demonstrated in microorganisms such as Geotrichum candidum strain GcG. This method utilizes methanethiol (CH₃SH) and acetyl-CoA as substrates, with the reaction catalyzed by a thioester synthase enzyme . The process involves the nucleophilic attack of the thiol group of methanethiol on the carbonyl carbon of acetyl-CoA, forming MTA and releasing free coenzyme A (CoA-SH):
3\text{SH} + \text{Acetyl-CoA} \rightarrow \text{CH}3\text{C(O)SCH}_3 + \text{CoA-SH} \quad
Key factors influencing this reaction include:
-
pH Dependence : Optimal activity occurs at neutral to slightly acidic conditions (pH 6.0–7.0), as alkaline conditions favor spontaneous non-enzymatic synthesis .
-
Temperature Sensitivity : Enzyme activity peaks at 30–35°C, while temperatures above 40°C lead to denaturation .
-
Substrate Length : Enzymatic specificity is highest for acetyl-CoA (C2); longer acyl-CoA chains (C3–C6) undergo spontaneous thioesterification without enzyme involvement .
Spontaneous vs. Catalyzed Synthesis
At pH > 8.0 or elevated temperatures (>50°C), MTA forms spontaneously via nucleophilic acyl substitution between methanethiol and acetyl-CoA. However, this non-enzymatic route produces lower yields (15–20%) compared to enzymatic methods (60–70%) .
Chemical Synthesis via Acetic Anhydride and Sodium Thiomethoxide
Reaction Mechanism and Conditions
A widely used chemical method involves the reaction of acetic anhydride ((CH₃CO)₂O) with sodium thiomethoxide (CH₃SNa) . This exothermic process proceeds as follows:
3\text{CO)}2\text{O} + \text{CH}3\text{SNa} \rightarrow \text{CH}3\text{C(O)SCH}3 + \text{CH}3\text{COONa} \quad
Optimization Parameters :
-
Solvent Choice : Anhydrous tetrahydrofuran (THF) or diethyl ether enhances yield by minimizing hydrolysis .
-
Stoichiometry : A 1:1 molar ratio of acetic anhydride to sodium thiomethoxide achieves 85–90% conversion .
-
Purification : Distillation under reduced pressure (50–60°C at 20 mmHg) isolates MTA as a colorless liquid .
Industrial-Scale Production
Large-scale synthesis employs continuous-flow reactors to control exothermicity. Key suppliers such as Hebei Yanxi Chemical Co. and JINING XINHE CHEMICAL utilize this method, producing MTA with >98% purity for flavor and fragrance applications .
Biotechnological Production Using Brewer’s Yeast
Fermentation-Based Synthesis
Saccharomyces cerevisiae converts methyl mercaptan (CH₃SH) into MTA under aerobic conditions. In a study by Matsui and Amaha, brewer’s yeast produced 98 mg/L of MTA from 500 mg/L of CH₃SH in 72 hours . Key findings include:
-
Substrate Inhibition : CH₃SH concentrations >1 g/L suppressed yeast growth and halted MTA production .
-
Resting Cell Efficiency : Washed yeast cells in stationary phase synthesized MTA at 2.6 mg/L/hr without additional nutrients, suggesting a cell-bound enzyme system .
Pathway Elucidation
The yeast-mediated process likely involves acetylation of CH₃SH by acetyl-CoA, analogous to the Geotrichum pathway. Metal chelators like EDTA and o-phenanthroline inhibited MTA synthesis by 80–90%, implicating a metalloenzyme in the acetylation step .
Electrochemical CO₂ Fixation on Nickel Sulfide Catalysts
Geoelectrochemical Synthesis
A novel method using nickel sulfide (NiS) catalysts achieves MTA synthesis from carbon monoxide (CO) and methanethiol at room temperature and neutral pH . The proposed mechanism involves:
-
C–S Bond Cleavage : Ni⁰ sites facilitate reductive cleavage of CH₃SH, generating methyl radicals.
-
Acetyl Formation : CO adsorbs onto adjacent Ni⁰, forming acetyl intermediates.
-
Thioesterification : Acetyl-Ni⁰ undergoes oxidative coupling with CH₃SH, yielding MTA .
Performance Metrics :
-
Yield : 35% based on CO input.
-
Byproducts : Dimethyl sulfide (10–15%) and methane (5–8%) form via competing pathways .
Comparative Analysis of Synthesis Methods
Method | Yield | Conditions | Catalyst | Scalability |
---|---|---|---|---|
Enzymatic (G. candidum) | 60–70% | 30–35°C, pH 6.0–7.0 | Thioester synthase | Lab-scale |
Chemical (Acetic Anhydride) | 85–90% | 25°C, anhydrous solvent | None | Industrial |
Yeast Fermentation | 20–25% | 20°C, aerobic | S. cerevisiae | Pilot-scale |
Electrochemical (NiS) | 35% | RT, neutral pH | NiS nanoparticles | Experimental |
Scientific Research Applications
Food Science
Flavor Profile and Aroma
S-Methyl thioacetate is known for its distinctive sulfurous and cabbage-like aroma, contributing to the flavor profiles of several foods. It has been detected in various products including fruits, coffee, and dairy items . Its presence in foods suggests potential applications as a flavoring agent or biomarker for food consumption.
Case Study: Wine Aging
Research has shown that this compound evolves during the aging process of wines, particularly in oak barrels. This study highlighted its role in developing the complex aromas associated with aged wines, indicating its significance in the wine industry .
Biochemical Applications
Enzymatic Synthesis
this compound can be synthesized enzymatically from methanethiol and acetyl-CoA through microbial processes. For example, Geotrichum candidum has been shown to produce this compound under specific pH and temperature conditions . This enzymatic pathway emphasizes its potential use in biotechnological applications for producing flavor compounds.
Thioester Formation Studies
Recent studies have explored the synthesis of thioesters like this compound through geoelectrochemical processes. Nickel sulfide (NiS) has been demonstrated to facilitate the formation of this compound from carbon monoxide and methanethiol under simulated primordial conditions, suggesting insights into prebiotic chemistry and the origins of life .
Environmental Chemistry
Biomarker Potential
Given its detection in various food sources, this compound could serve as a biomarker for dietary intake studies. Its presence in human metabolism indicates potential applications in nutritional epidemiology and health studies .
Chemical Research
Hydrolysis Mechanisms
Studies on the hydrolysis of this compound have provided insights into reaction mechanisms involving thiol esters. Theoretical models indicate different pathways for hydrolysis in gas versus solution phases, which could inform synthetic strategies in organic chemistry .
Production from Fatty Acids
Research involving Brevibacterium species has shown that these bacteria can produce this compound from fatty acids and branched-chain amino acids. This finding opens avenues for exploring microbial fermentation processes to generate flavor compounds used in food production .
Summary Table of Applications
Application Area | Description | Key Findings/Case Studies |
---|---|---|
Food Science | Contributes to flavor profiles; detected in fruits and dairy products | Evolved during wine aging; significant for aroma development |
Biochemical Applications | Enzymatic synthesis via microbial pathways | Produced by Geotrichum candidum under specific conditions |
Environmental Chemistry | Potential biomarker for dietary studies | Detected in human metabolism; implications for health research |
Chemical Research | Insights into hydrolysis mechanisms | Different pathways observed in gas vs solution phases |
Production from Fatty Acids | Microbial production from fatty acids and amino acids | Identified pathways for producing flavor compounds |
Mechanism of Action
The mechanism of action of S-Methyl thioacetate involves its interaction with various molecular targets. In biological systems, it can undergo enzymatic transformations, leading to the formation of metabolites that participate in metabolic pathways. The sulfur atom in the compound plays a crucial role in its reactivity and interaction with other molecules .
Comparison with Similar Compounds
Structural and Functional Analogues
2.1.1. S-Methyl Thioisovalerate and S-Methyl Thioisobutyrate
- Structure : Both are branched-chain thioesters derived from L-leucine catabolism. S-Methyl thioisovalerate (C₅H₁₀OS) has an isovaleryl group, while S-Methyl thioisobutyrate (C₅H₁₀OS) features an isobutyryl chain, compared to the linear acetyl group in S-methyl thioacetate (C₃H₆OS) .
- Biosynthesis: All three are produced by Brevibacterium spp. via transamination of L-leucine and subsequent acyl-CoA intermediates. However, this compound is additionally formed from exogenous fatty acids in Micrococcaceae under acidic conditions .
- Applications : S-Methyl thioisovalerate and thioisobutyrate are less studied but likely contribute to cheese ripening aromas. This compound has broader roles in food flavoring and antimicrobial activity .
2.1.2. Dimethyl Disulfide (DMDS)
- Structure : DMDS (CH₃SSCH₃) is a disulfide, lacking the carbonyl group of thioesters.
- Reactivity : DMDS is less reactive in acyl-transfer reactions but more volatile. Both compounds exhibit antifungal activity, though DMDS is more potent and commercially used as a soil fumigant .
- Biosynthesis : DMDS arises from methanethiol oxidation, whereas this compound requires methanethiol condensation with acetyl-CoA or abiotic synthesis .
Physicochemical and Reactivity Profiles
Property | This compound | S-Methyl Thiopropionate | Acetyl-CoA |
---|---|---|---|
Molecular Formula | C₃H₆OS | C₄H₈OS | C₂₃H₃₈N₇O₁₇P₃S |
Carbonyl Reactivity | Ketone-like (no C=O π-bonding) | Similar to thioacetate | High biochemical reactivity (acyl transfer) |
Exchange Constant (kₑₓ) | 1.7 M⁻¹s⁻¹ | Not reported | ~10³–10⁶ M⁻¹s⁻¹ |
Key Applications | Flavoring, antimicrobial | Flavor additive | Metabolic cofactor |
- Reactivity Notes: this compound’s kₑₓ is lower than phenyl thioesters (120 M⁻¹s⁻¹) but comparable to alkyl thioesters like S-methyl thiobutyrate, indicating moderate stability in aqueous solutions . Its cis conformation dominates (>95% abundance), unlike trans-configured esters, reducing steric hindrance in interactions .
Antimicrobial Efficacy
Organism | This compound Effect | Dimethyl Disulfide (DMDS) Effect |
---|---|---|
Rhizoctonia solani | Hyphae radius reduced by 40% | Hyphae radius reduced by 60% |
Pythium ultimum | Growth inhibition at 50 ppm | Complete inhibition at 20 ppm |
Root-knot nematodes | LC₅₀: 15 ppm | LC₅₀: 5 ppm |
- This compound’s lower volatility compared to DMDS makes it less effective in soil applications but suitable for controlled-release formulations .
Flavor and Odor Profiles
Biological Activity
S-Methyl thioacetate (MTA), a member of the thioester family, has garnered interest due to its biological activity and potential applications in various fields, including biochemistry and food science. This article explores the synthesis, enzymatic activity, metabolic pathways, and potential health implications of this compound, supported by research findings and data tables.
This compound is chemically characterized by the formula and is known for its distinctive cabbage and cheese-like aroma. It can be synthesized through various methods, including enzymatic reactions using microorganisms like Geotrichum candidum or through chemical reactions involving methanethiol and acetyl-CoA.
Property | Value |
---|---|
IUPAC Name | 1-(methylsulfanyl)ethan-1-one |
CAS Registry Number | 1534-08-3 |
Molecular Weight | 90.144 g/mol |
Structure | Structure |
Enzymatic Synthesis
Research indicates that this compound can be synthesized enzymatically in Geotrichum candidum using methanethiol and acetyl-CoA as substrates. The synthesis is influenced by pH and temperature, with spontaneous reactions predominating for longer-chain acyl-CoA compounds (C3 to C6) while short-chain synthesis relies more on enzymatic pathways .
Case Studies
- Enzymatic vs. Spontaneous Formation : A study demonstrated that the synthesis of this compound from methanethiol and acetyl-CoA was significantly affected by environmental conditions. Enzymatic reactions were favored at lower pH levels, while spontaneous reactions were more prevalent at higher temperatures .
- Metabolic Pathways : In metabolic studies, this compound has been shown to play a role in cellular metabolism similar to that of acetyl-CoA, suggesting its potential as a metabolic precursor in biochemical pathways .
Hydrolysis and Stability
The stability of this compound under various conditions has been investigated through quantum mechanical calculations. These studies reveal that hydrolysis can occur via different mechanisms depending on the environment (gas vs. solution phase), affecting its reactivity and biological availability .
Table 2: Hydrolysis Mechanisms of this compound
Phase | Mechanism Type | Description |
---|---|---|
Gas Phase | Concerted | S(N)2 mechanism observed |
Solution Phase | Stepwise | Nucleophilic attack leading to tetrahedral intermediate |
Health Implications
This compound has been detected in various food products, including dairy and onion-family vegetables, suggesting its role as a flavor compound and potential biomarker for food consumption. Its presence in food items raises questions about health effects, although specific bioactivities remain under-researched .
Chemical Reactions Analysis
Geoelectrochemical CO₂ Fixation
-
Mechanism : CO and methanethiol react on NiS surfaces with adjacent Ni⁰ sites. One Ni⁰ cleaves the C–S bond of methanethiol, while another facilitates acetyl-Ni⁰ formation, followed by oxidative thiolysis to MTA .
Hydrolysis and Thiol-Thioester Exchange
MTA undergoes hydrolysis and exchange reactions, influenced by pH and catalysts:
Hydrolysis
-
Base-Mediated Hydrolysis : Follows second-order kinetics with at high pH .
-
Acid-Catalyzed Hydrolysis : Dominates below pH 6, with slower rates compared to base-mediated pathways .
Thiol-Thioester Exchange
-
Reaction : MTA reacts with thiols (e.g., mercaptoethanesulfonate) via nucleophilic substitution.
Reaction Type | Conditions | Rate Constant |
---|---|---|
Base hydrolysis | pH 9–12 | |
Thiol exchange | pH 6, 70°C |
Role in Prebiotic and Metabolic Pathways
MTA is implicated in primordial metabolism and modern biochemical processes:
Prebiotic Analog of Acetyl-CoA
-
MTA serves as a precursor to acetyl-CoA in simulated prebiotic conditions, formed via Ni/Fe-sulfide catalysts .
-
Iron-Sulfur Clusters : Concurrently forms FeS nanoparticles during thioester synthesis, relevant to early metabolic pathways .
Microbial Metabolism
-
Brevibacterium spp. : Convert branched-chain amino acids (e.g., leucine) to MTA via acyl-CoA intermediates .
-
Cheese Aroma : Contributes sulfurous notes in fermented products, with Geotrichum candidum as a key producer .
Quantum Chemical Insights
-
Reactivity : Lacks resonance stabilization compared to oxygen esters, increasing susceptibility to nucleophilic attack .
-
Barrier to Rotation : Lower rotational barrier (~2 kcal/mol) than methyl acetate, enhancing conformational flexibility .
Key Mechanistic Features
Q & A
Q. Basic: What laboratory synthesis methods are available for S-methyl thioacetate?
This compound is commonly synthesized via a "one-pot" reaction between methyl chlorothiolformate and carboxylic acids under controlled conditions . This method generates a library of thioesters, with this compound typically present in lower molar concentrations (~0.8%) due to volatility losses during extraction. Gas chromatography (GC) with flame ionization detection (FID) or sulfur chemiluminescence detection (SCD) is recommended for quantification and impurity analysis, such as identifying S,S-dimethyldithiocarbonate .
Q. Advanced: How can synthesis protocols be optimized to reduce impurities like S,S-dimethyldithiocarbonate?
Impurity formation is influenced by reaction stoichiometry, solvent choice, and extraction efficiency. Lowering reaction temperatures and using inert atmospheres can minimize side reactions. Fractional distillation or preparative GC may enhance purity, though volatility of this compound complicates recovery. Computational modeling (e.g., density functional theory) can predict reactive intermediates to refine reaction pathways .
Q. Basic: What spectroscopic techniques characterize this compound’s structure?
Gas electron diffraction (GED) and microwave spectroscopy have been used to determine its molecular geometry, revealing a planar C(O)S moiety and rotational barriers influencing conformational stability . Infrared (IR) and Raman spectroscopy identify vibrational modes associated with the thioester group (C=O stretch at ~1700 cm⁻¹, C-S stretch at ~650 cm⁻¹), critical for comparing reactivity with oxygen analogs .
Q. Advanced: How does conformational analysis inform this compound’s biochemical interactions?
The molecule’s planar conformation and electron delocalization in the thioester bond mimic acetyl-CoA’s reactivity, enabling studies on enzyme-substrate interactions (e.g., acetyltransferases). Computational models (ab initio molecular orbital calculations) correlate structural flexibility with nucleophilic susceptibility, aiding in designing enzyme inhibitors or biomimetic catalysts .
Q. Basic: What are the antimicrobial properties of this compound?
This compound exhibits antifungal activity against Pythium ultimum and Rhizoctonia solani, reducing hyphal growth by 40–60% at 100 ppm. Synergy with dimethyl disulfide (DMDS) enhances efficacy, suggesting combinatorial volatile organic compound (VOC) treatments as a plant pathogen control strategy .
Q. Advanced: How should experimental designs account for contradictory data in antimicrobial studies?
Contradictions may arise from VOC volatility, microbial strain variability, or environmental factors (e.g., pH, temperature). Rigorous controls (e.g., sealed incubation chambers for VOCs) and statistical validation (one-way ANOVA with post hoc t-tests) are essential. Dose-response curves and time-lapse imaging (as in Fig. 4B ) improve reproducibility.
Q. Basic: What safety protocols are critical when handling this compound?
Due to its flammability (flash point: 12°C) and irritancy, use fume hoods, explosion-proof equipment, and personal protective gear (nitrile gloves, goggles). Immediate decontamination with soap/water is required for skin contact. Store under nitrogen at –20°C to prevent degradation .
Q. Advanced: How do enzymatic pathways in microorganisms synthesize this compound?
Geotrichum candidum produces this compound via enzymatic condensation of methanethiol and acetyl-CoA. pH-dependent activity (optimal at pH 7) and substrate specificity (preference for C2–C4 acyl-CoA derivatives) influence yield. Metabolomic profiling (LC-MS/MS) and gene knockout studies can identify key enzymes (e.g., thioesterases) for pathway engineering .
Q. Basic: How is this compound analyzed in complex matrices (e.g., microbial cultures)?
Reverse-phase HPLC with log k(w) (lipophilicity) coefficients predicts partitioning in lipid phases (e.g., triolein). Solid-phase microextraction (SPME) coupled with GC-MS is effective for trace VOC detection in biological samples .
Q. Advanced: What strategies resolve discrepancies in physicochemical property measurements (e.g., log P)?
Inter-laboratory variability arises from solvent purity, temperature, and column calibration. Standardized protocols (e.g., OECD 117 shake-flask method) and cross-validation with computational models (e.g., COSMO-RS) improve consistency. Collaborative ring trials and open-data repositories enhance reliability .
Properties
IUPAC Name |
S-methyl ethanethioate | |
---|---|---|
Source | PubChem | |
URL | https://pubchem.ncbi.nlm.nih.gov | |
Description | Data deposited in or computed by PubChem | |
InChI |
InChI=1S/C3H6OS/c1-3(4)5-2/h1-2H3 | |
Source | PubChem | |
URL | https://pubchem.ncbi.nlm.nih.gov | |
Description | Data deposited in or computed by PubChem | |
InChI Key |
OATSQCXMYKYFQO-UHFFFAOYSA-N | |
Source | PubChem | |
URL | https://pubchem.ncbi.nlm.nih.gov | |
Description | Data deposited in or computed by PubChem | |
Canonical SMILES |
CC(=O)SC | |
Source | PubChem | |
URL | https://pubchem.ncbi.nlm.nih.gov | |
Description | Data deposited in or computed by PubChem | |
Molecular Formula |
C3H6OS | |
Source | PubChem | |
URL | https://pubchem.ncbi.nlm.nih.gov | |
Description | Data deposited in or computed by PubChem | |
DSSTOX Substance ID |
DTXSID3073264 | |
Record name | Ethanethioic acid, S-methyl ester | |
Source | EPA DSSTox | |
URL | https://comptox.epa.gov/dashboard/DTXSID3073264 | |
Description | DSSTox provides a high quality public chemistry resource for supporting improved predictive toxicology. | |
Molecular Weight |
90.15 g/mol | |
Source | PubChem | |
URL | https://pubchem.ncbi.nlm.nih.gov | |
Description | Data deposited in or computed by PubChem | |
Physical Description |
Liquid, colourless to pale yellow liquid | |
Record name | S-Methyl thioacetate | |
Source | Human Metabolome Database (HMDB) | |
URL | http://www.hmdb.ca/metabolites/HMDB0031189 | |
Description | The Human Metabolome Database (HMDB) is a freely available electronic database containing detailed information about small molecule metabolites found in the human body. | |
Explanation | HMDB is offered to the public as a freely available resource. Use and re-distribution of the data, in whole or in part, for commercial purposes requires explicit permission of the authors and explicit acknowledgment of the source material (HMDB) and the original publication (see the HMDB citing page). We ask that users who download significant portions of the database cite the HMDB paper in any resulting publications. | |
Record name | S-Methyl thioacetate | |
Source | Joint FAO/WHO Expert Committee on Food Additives (JECFA) | |
URL | https://www.fao.org/food/food-safety-quality/scientific-advice/jecfa/jecfa-flav/details/en/c/112/ | |
Description | The flavoring agent databse provides the most recent specifications for flavorings evaluated by JECFA. | |
Explanation | Permission from WHO is not required for the use of WHO materials issued under the Creative Commons Attribution-NonCommercial-ShareAlike 3.0 Intergovernmental Organization (CC BY-NC-SA 3.0 IGO) licence. | |
Boiling Point |
95.00 to 96.00 °C. @ 760.00 mm Hg | |
Record name | S-Methyl thioacetate | |
Source | Human Metabolome Database (HMDB) | |
URL | http://www.hmdb.ca/metabolites/HMDB0031189 | |
Description | The Human Metabolome Database (HMDB) is a freely available electronic database containing detailed information about small molecule metabolites found in the human body. | |
Explanation | HMDB is offered to the public as a freely available resource. Use and re-distribution of the data, in whole or in part, for commercial purposes requires explicit permission of the authors and explicit acknowledgment of the source material (HMDB) and the original publication (see the HMDB citing page). We ask that users who download significant portions of the database cite the HMDB paper in any resulting publications. | |
Solubility |
soluble in oil and alcohol | |
Record name | S-Methyl thioacetate | |
Source | Joint FAO/WHO Expert Committee on Food Additives (JECFA) | |
URL | https://www.fao.org/food/food-safety-quality/scientific-advice/jecfa/jecfa-flav/details/en/c/112/ | |
Description | The flavoring agent databse provides the most recent specifications for flavorings evaluated by JECFA. | |
Explanation | Permission from WHO is not required for the use of WHO materials issued under the Creative Commons Attribution-NonCommercial-ShareAlike 3.0 Intergovernmental Organization (CC BY-NC-SA 3.0 IGO) licence. | |
Density |
0.817-0.825 | |
Record name | S-Methyl thioacetate | |
Source | Joint FAO/WHO Expert Committee on Food Additives (JECFA) | |
URL | https://www.fao.org/food/food-safety-quality/scientific-advice/jecfa/jecfa-flav/details/en/c/112/ | |
Description | The flavoring agent databse provides the most recent specifications for flavorings evaluated by JECFA. | |
Explanation | Permission from WHO is not required for the use of WHO materials issued under the Creative Commons Attribution-NonCommercial-ShareAlike 3.0 Intergovernmental Organization (CC BY-NC-SA 3.0 IGO) licence. | |
CAS No. |
1534-08-3 | |
Record name | S-Methyl thioacetate | |
Source | CAS Common Chemistry | |
URL | https://commonchemistry.cas.org/detail?cas_rn=1534-08-3 | |
Description | CAS Common Chemistry is an open community resource for accessing chemical information. Nearly 500,000 chemical substances from CAS REGISTRY cover areas of community interest, including common and frequently regulated chemicals, and those relevant to high school and undergraduate chemistry classes. This chemical information, curated by our expert scientists, is provided in alignment with our mission as a division of the American Chemical Society. | |
Explanation | The data from CAS Common Chemistry is provided under a CC-BY-NC 4.0 license, unless otherwise stated. | |
Record name | Methyl thioacetate | |
Source | ChemIDplus | |
URL | https://pubchem.ncbi.nlm.nih.gov/substance/?source=chemidplus&sourceid=0001534083 | |
Description | ChemIDplus is a free, web search system that provides access to the structure and nomenclature authority files used for the identification of chemical substances cited in National Library of Medicine (NLM) databases, including the TOXNET system. | |
Record name | Ethanethioic acid, S-methyl ester | |
Source | EPA DSSTox | |
URL | https://comptox.epa.gov/dashboard/DTXSID3073264 | |
Description | DSSTox provides a high quality public chemistry resource for supporting improved predictive toxicology. | |
Record name | S-METHYL THIOACETATE | |
Source | FDA Global Substance Registration System (GSRS) | |
URL | https://gsrs.ncats.nih.gov/ginas/app/beta/substances/PF2D4MWX79 | |
Description | The FDA Global Substance Registration System (GSRS) enables the efficient and accurate exchange of information on what substances are in regulated products. Instead of relying on names, which vary across regulatory domains, countries, and regions, the GSRS knowledge base makes it possible for substances to be defined by standardized, scientific descriptions. | |
Explanation | Unless otherwise noted, the contents of the FDA website (www.fda.gov), both text and graphics, are not copyrighted. They are in the public domain and may be republished, reprinted and otherwise used freely by anyone without the need to obtain permission from FDA. Credit to the U.S. Food and Drug Administration as the source is appreciated but not required. | |
Record name | S-Methyl thioacetate | |
Source | Human Metabolome Database (HMDB) | |
URL | http://www.hmdb.ca/metabolites/HMDB0031189 | |
Description | The Human Metabolome Database (HMDB) is a freely available electronic database containing detailed information about small molecule metabolites found in the human body. | |
Explanation | HMDB is offered to the public as a freely available resource. Use and re-distribution of the data, in whole or in part, for commercial purposes requires explicit permission of the authors and explicit acknowledgment of the source material (HMDB) and the original publication (see the HMDB citing page). We ask that users who download significant portions of the database cite the HMDB paper in any resulting publications. | |
Retrosynthesis Analysis
AI-Powered Synthesis Planning: Our tool employs the Template_relevance Pistachio, Template_relevance Bkms_metabolic, Template_relevance Pistachio_ringbreaker, Template_relevance Reaxys, Template_relevance Reaxys_biocatalysis model, leveraging a vast database of chemical reactions to predict feasible synthetic routes.
One-Step Synthesis Focus: Specifically designed for one-step synthesis, it provides concise and direct routes for your target compounds, streamlining the synthesis process.
Accurate Predictions: Utilizing the extensive PISTACHIO, BKMS_METABOLIC, PISTACHIO_RINGBREAKER, REAXYS, REAXYS_BIOCATALYSIS database, our tool offers high-accuracy predictions, reflecting the latest in chemical research and data.
Strategy Settings
Precursor scoring | Relevance Heuristic |
---|---|
Min. plausibility | 0.01 |
Model | Template_relevance |
Template Set | Pistachio/Bkms_metabolic/Pistachio_ringbreaker/Reaxys/Reaxys_biocatalysis |
Top-N result to add to graph | 6 |
Feasible Synthetic Routes
Disclaimer and Information on In-Vitro Research Products
Please be aware that all articles and product information presented on BenchChem are intended solely for informational purposes. The products available for purchase on BenchChem are specifically designed for in-vitro studies, which are conducted outside of living organisms. In-vitro studies, derived from the Latin term "in glass," involve experiments performed in controlled laboratory settings using cells or tissues. It is important to note that these products are not categorized as medicines or drugs, and they have not received approval from the FDA for the prevention, treatment, or cure of any medical condition, ailment, or disease. We must emphasize that any form of bodily introduction of these products into humans or animals is strictly prohibited by law. It is essential to adhere to these guidelines to ensure compliance with legal and ethical standards in research and experimentation.