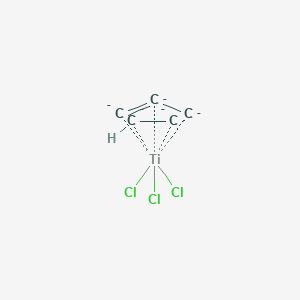
Trichloro-pi-cyclopentadianyltitanium
- Click on QUICK INQUIRY to receive a quote from our team of experts.
- With the quality product at a COMPETITIVE price, you can focus more on your research.
Overview
Description
It is a moisture-sensitive orange solid that adopts a piano stool geometry . This compound is notable for its electrophilic nature and its ability to form various complexes with other ligands.
Preparation Methods
Synthetic Routes and Reaction Conditions: Trichloro-pi-cyclopentadianyltitanium is typically synthesized through the reaction of titanocene dichloride with titanium tetrachloride:
(C5H5)2TiCl2+TiCl4→2(C5H5)TiCl3
This reaction is carried out under controlled conditions to ensure the formation of the desired product .
Industrial Production Methods: Industrial production of this compound involves similar synthetic routes but on a larger scale. The process requires precise control of temperature and pressure to maintain the integrity of the compound. The use of high-purity reagents and solvents is crucial to avoid contamination and ensure high yield.
Types of Reactions:
- this compound can be reduced using zinc powder to form polymeric titanium (III) derivatives:
Reduction: (C5H5)TiCl3+0.5Zn→1/n[(C5H5)TiCl2]n+ZnCl2
A similar reduction can be achieved using cobaltocene .Substitution: The compound readily forms alkoxide complexes upon treatment with alcohols.
Common Reagents and Conditions:
Zinc Powder: Used for reduction reactions.
Cobaltocene: Another reducing agent.
Alcohols: For forming alkoxide complexes.
Phosphine Ligands: For forming adducts.
Major Products:
Polymeric Titanium (III) Derivatives: Formed through reduction.
Alkoxide Complexes: Formed through substitution with alcohols.
Phosphine Adducts: Formed through substitution with phosphine ligands.
Scientific Research Applications
Trichloro-pi-cyclopentadianyltitanium has a wide range of applications in scientific research:
Organic Synthesis: The compound’s electrophilic nature makes it useful in organic synthesis for forming various complexes and intermediates.
Material Science: Its unique properties are exploited in the development of new materials with specific electronic and structural characteristics.
Electrochemical Studies: The compound’s electrochemical behavior has been studied using techniques like polarography and cyclic voltammetry.
Mechanism of Action
The mechanism by which trichloro-pi-cyclopentadianyltitanium exerts its effects involves its electrophilic nature, which allows it to readily form complexes with various ligands. The compound’s molecular targets include alcohols, phosphine ligands, and reducing agents like zinc and cobaltocene. These interactions lead to the formation of various products, including alkoxide complexes, phosphine adducts, and polymeric titanium (III) derivatives .
Comparison with Similar Compounds
- (Cyclopentadienyl)zirconium trichloride
- (Pentamethylcyclopentadienyl)titanium trichloride
- (Indenyl)titanium trichloride
Comparison: Trichloro-pi-cyclopentadianyltitanium is unique due to its specific electrophilic properties and its ability to form a wide range of complexes. Compared to its analogs, such as (Cyclopentadienyl)zirconium trichloride and (Pentamethylcyclopentadienyl)titanium trichloride, it exhibits distinct reactivity patterns and stability under various conditions .
Properties
CAS No. |
1270-98-0 |
---|---|
Molecular Formula |
C5H5Cl3Ti |
Molecular Weight |
219.31 g/mol |
IUPAC Name |
cyclopenta-1,3-diene;titanium(4+);trichloride |
InChI |
InChI=1S/C5H5.3ClH.Ti/c1-2-4-5-3-1;;;;/h1-5H;3*1H;/q-1;;;;+4/p-3 |
InChI Key |
QOXHZZQZTIGPEV-UHFFFAOYSA-K |
SMILES |
[CH-]1[C-]=[C-][C-]=[C-]1.Cl[Ti](Cl)Cl |
Canonical SMILES |
[CH-]1C=CC=C1.[Cl-].[Cl-].[Cl-].[Ti+4] |
Origin of Product |
United States |
Disclaimer and Information on In-Vitro Research Products
Please be aware that all articles and product information presented on BenchChem are intended solely for informational purposes. The products available for purchase on BenchChem are specifically designed for in-vitro studies, which are conducted outside of living organisms. In-vitro studies, derived from the Latin term "in glass," involve experiments performed in controlled laboratory settings using cells or tissues. It is important to note that these products are not categorized as medicines or drugs, and they have not received approval from the FDA for the prevention, treatment, or cure of any medical condition, ailment, or disease. We must emphasize that any form of bodily introduction of these products into humans or animals is strictly prohibited by law. It is essential to adhere to these guidelines to ensure compliance with legal and ethical standards in research and experimentation.