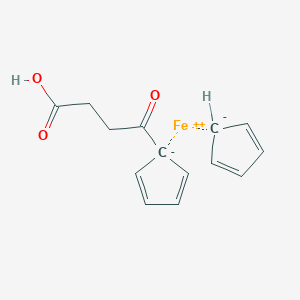
4-Ferrocenyl-4-oxobutyric Acid
Overview
Description
4-Ferrocenyl-4-oxobutyric Acid is a useful research compound. Its molecular formula is C14H14FeO3 and its molecular weight is 286.10 g/mol. The purity is usually 95%.
BenchChem offers high-quality this compound suitable for many research applications. Different packaging options are available to accommodate customers' requirements. Please inquire for more information about this compound including the price, delivery time, and more detailed information at info@benchchem.com.
Scientific Research Applications
Antibiotic Derivatives : Patra et al. (2011) synthesized enantiomers of a C6–C7 ferrocene-fused platensimycin derivative starting from 4-ferrocenyl-4-oxobutyric acid. These compounds showed potential as antibiotics against various Gram-positive and Gram-negative bacterial strains (Patra et al., 2011).
Electrochemical Properties : Paolucci et al. (2005) studied the electronic properties of ferrocenyl-based π-conjugated complexes, indicating their potential use in the preparation of redox-active complexes (Paolucci et al., 2005).
Nonlinear Optical Properties : Li et al. (2003) explored the nonlinear optical properties of novel ferrocenyl complexes, which exhibited strong self-focusing effects, suggesting their utility in optical applications (Li et al., 2003).
Electrochemistry : Hildebrandt et al. (2010) synthesized a supercrowded tetraferrocenylthiophene and investigated its electrochemical properties, highlighting its potential in electron-transfer applications (Hildebrandt et al., 2010).
Oxidative DNA Damage Assay : Nawaz et al. (2009) synthesized Schiff-base esters of ferrocenyl aniline and evaluated their antitumor, antioxidant, and DNA protective activities (Nawaz et al., 2009).
Chemosensors : Kumar et al. (2013) developed multichannel signaling receptors for metal ions using 1-(2-pyridyl)-3-ferrocenylpyrazolines, indicating their potential in the selective detection of metal ions (Kumar et al., 2013).
Biomedical Applications : Swarts et al. (1994) synthesized polymer-ferrocene conjugates for potential use in biomedical applications, particularly in cancer research (Swarts et al., 1994).
Mechanism of Action
Safety and Hazards
While specific safety data for 4-Ferrocenyl-4-oxobutyric Acid is not available, it’s important to handle all chemicals with care. General safety measures include avoiding breathing dust/fume/gas/mist/vapors/spray, using only outdoors or in a well-ventilated area, and wearing protective gloves/protective clothing/eye protection/face protection .
Future Directions
Ferrocene-based compounds, including 4-Ferrocenyl-4-oxobutyric Acid, have many applications in diverse scientific disciplines, including in polymer chemistry as redox dynamic polymers and dendrimers, in materials science as bioreceptors, and in pharmacology, biochemistry, electrochemistry, and nonlinear optics . The future of this compound and similar compounds lies in further exploring these applications and developing new ones.
Biochemical Analysis
Biochemical Properties
Ferrocene-based compounds are known to have applications in diverse scientific disciplines, including polymer chemistry as redox dynamic polymers and dendrimers, in materials science as bioreceptors, and in pharmacology, biochemistry, electrochemistry, and nonlinear optics .
Cellular Effects
Ferrocene-based compounds have been studied for their effects on cancer cells, particularly breast and prostate cancers . The proliferative/anti-proliferative effects of compounds based on steroidal and non-steroidal endocrine modulators have been extensively explored in vitro .
Molecular Mechanism
Ferrocene-based compounds have been used in the development of anticancer, antiviral, and antimicrobial drugs . Ferrocene derivatives have been used to synthesize a wide range of materials, including polymers, liquid crystals, and nanoparticles, with diverse electronic and magnetic properties .
Temporal Effects in Laboratory Settings
Ferrocene-based compounds have been used in the development of anticancer, antiviral, and antimicrobial drugs .
Dosage Effects in Animal Models
Ferrocene-based compounds have been studied for their effects on cancer cells, particularly breast and prostate cancers .
Metabolic Pathways
Ferrocene-based compounds have been used in the development of anticancer, antiviral, and antimicrobial drugs .
Transport and Distribution
Ferrocene-based compounds have been used in the development of anticancer, antiviral, and antimicrobial drugs .
Subcellular Localization
Ferrocene-based compounds have been used in the development of anticancer, antiviral, and antimicrobial drugs .
Properties
IUPAC Name |
cyclopenta-1,3-diene;1-cyclopenta-2,4-dien-1-ylidene-4-hydroxy-4-oxobutan-1-olate;iron(2+) | |
---|---|---|
Details | Computed by Lexichem TK 2.7.0 (PubChem release 2021.05.07) | |
Source | PubChem | |
URL | https://pubchem.ncbi.nlm.nih.gov | |
Description | Data deposited in or computed by PubChem | |
InChI |
InChI=1S/C9H10O3.C5H5.Fe/c10-8(5-6-9(11)12)7-3-1-2-4-7;1-2-4-5-3-1;/h1-4,10H,5-6H2,(H,11,12);1-5H;/q;-1;+2/p-1 | |
Details | Computed by InChI 1.0.6 (PubChem release 2021.05.07) | |
Source | PubChem | |
URL | https://pubchem.ncbi.nlm.nih.gov | |
Description | Data deposited in or computed by PubChem | |
InChI Key |
DJYFWOWHTBEOSI-UHFFFAOYSA-M | |
Details | Computed by InChI 1.0.6 (PubChem release 2021.05.07) | |
Source | PubChem | |
URL | https://pubchem.ncbi.nlm.nih.gov | |
Description | Data deposited in or computed by PubChem | |
Canonical SMILES |
[CH-]1C=CC=C1.C1=CC(=C(CCC(=O)O)[O-])C=C1.[Fe+2] | |
Details | Computed by OEChem 2.3.0 (PubChem release 2021.05.07) | |
Source | PubChem | |
URL | https://pubchem.ncbi.nlm.nih.gov | |
Description | Data deposited in or computed by PubChem | |
Molecular Formula |
C14H14FeO3 | |
Details | Computed by PubChem 2.1 (PubChem release 2021.05.07) | |
Source | PubChem | |
URL | https://pubchem.ncbi.nlm.nih.gov | |
Description | Data deposited in or computed by PubChem | |
DSSTOX Substance ID |
DTXSID60570698 | |
Record name | Iron(2+) 2-(3-carboxypropanoyl)cyclopenta-2,4-dien-1-ide cyclopenta-2,4-dien-1-ide (1/1/1) | |
Source | EPA DSSTox | |
URL | https://comptox.epa.gov/dashboard/DTXSID60570698 | |
Description | DSSTox provides a high quality public chemistry resource for supporting improved predictive toxicology. | |
Molecular Weight |
286.10 g/mol | |
Details | Computed by PubChem 2.1 (PubChem release 2021.05.07) | |
Source | PubChem | |
URL | https://pubchem.ncbi.nlm.nih.gov | |
Description | Data deposited in or computed by PubChem | |
CAS No. |
1291-72-1 | |
Record name | Iron(2+) 2-(3-carboxypropanoyl)cyclopenta-2,4-dien-1-ide cyclopenta-2,4-dien-1-ide (1/1/1) | |
Source | EPA DSSTox | |
URL | https://comptox.epa.gov/dashboard/DTXSID60570698 | |
Description | DSSTox provides a high quality public chemistry resource for supporting improved predictive toxicology. | |
Retrosynthesis Analysis
AI-Powered Synthesis Planning: Our tool employs the Template_relevance Pistachio, Template_relevance Bkms_metabolic, Template_relevance Pistachio_ringbreaker, Template_relevance Reaxys, Template_relevance Reaxys_biocatalysis model, leveraging a vast database of chemical reactions to predict feasible synthetic routes.
One-Step Synthesis Focus: Specifically designed for one-step synthesis, it provides concise and direct routes for your target compounds, streamlining the synthesis process.
Accurate Predictions: Utilizing the extensive PISTACHIO, BKMS_METABOLIC, PISTACHIO_RINGBREAKER, REAXYS, REAXYS_BIOCATALYSIS database, our tool offers high-accuracy predictions, reflecting the latest in chemical research and data.
Strategy Settings
Precursor scoring | Relevance Heuristic |
---|---|
Min. plausibility | 0.01 |
Model | Template_relevance |
Template Set | Pistachio/Bkms_metabolic/Pistachio_ringbreaker/Reaxys/Reaxys_biocatalysis |
Top-N result to add to graph | 6 |
Feasible Synthetic Routes
Q1: What is the significance of using 4-Ferrocenyl-4-oxobutyric acid in synthesizing these novel platensimycin derivatives?
A1: this compound serves as a crucial starting material in the multi-step synthesis of the ferrocene-fused platensimycin derivative, (S,SP)-2b []. The researchers utilized its structure to introduce a ferrocene moiety into the target molecule. This is significant because the ferrocene group is thought to mimic the spatial occupancy of the tetracyclic cage found in naturally occurring platensimycin within the binding site of the target enzyme, FabF []. This suggests that incorporating ferrocene could be a viable strategy for developing new antibacterial agents.
Q2: Can you elaborate on the key step in the synthesis process where this compound plays a central role?
A2: The synthetic route employed a highly diastereoselective Michael addition reaction. In this crucial step, optically active planar-chiral ferrocene-fused cyclohexanone derivatives (derived from this compound) reacted with an acrylate ester []. This reaction enabled the researchers to build the core structure of the desired platensimycin derivative with high stereoselectivity, a critical factor for biological activity.
Disclaimer and Information on In-Vitro Research Products
Please be aware that all articles and product information presented on BenchChem are intended solely for informational purposes. The products available for purchase on BenchChem are specifically designed for in-vitro studies, which are conducted outside of living organisms. In-vitro studies, derived from the Latin term "in glass," involve experiments performed in controlled laboratory settings using cells or tissues. It is important to note that these products are not categorized as medicines or drugs, and they have not received approval from the FDA for the prevention, treatment, or cure of any medical condition, ailment, or disease. We must emphasize that any form of bodily introduction of these products into humans or animals is strictly prohibited by law. It is essential to adhere to these guidelines to ensure compliance with legal and ethical standards in research and experimentation.