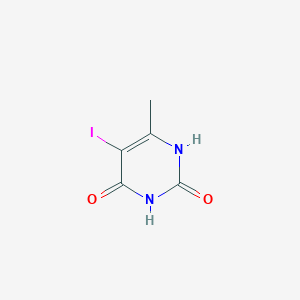
5-Iodo-6-methyluracil
Overview
Description
5-Iodo-6-methyluracil is a halogenated derivative of uracil, a pyrimidine nucleobase It is characterized by the presence of an iodine atom at the 5th position and a methyl group at the 6th position of the uracil ring
Preparation Methods
Synthetic Routes and Reaction Conditions: The primary method for synthesizing 5-iodo-6-methyluracil involves the oxidative halogenation of 6-methyluracil. This process typically uses elemental iodine and an oxidizing agent such as hydrogen peroxide or sodium nitrate. The reaction conditions often include an aqueous medium and controlled temperature to ensure the selective iodination at the 5th position .
Industrial Production Methods: While specific industrial production methods for this compound are not extensively documented, the general approach would involve scaling up the laboratory synthesis process. This would include optimizing the reaction conditions for higher yields and purity, as well as implementing efficient purification techniques such as recrystallization or chromatography.
Chemical Reactions Analysis
Types of Reactions: 5-Iodo-6-methyluracil undergoes various chemical reactions, including:
Substitution Reactions: The iodine atom can be replaced by other nucleophiles, such as bromine or chlorine, under appropriate conditions.
Oxidation and Reduction Reactions: The compound can participate in redox reactions, although specific examples are less documented.
Common Reagents and Conditions:
Iodination: Iodine and hydrogen peroxide in an aqueous medium.
Bromination: Bromine or N-bromosuccinimide in the presence of a catalyst.
Chlorination: Elemental chlorine or N-chlorosuccinimide.
Major Products:
5-Bromo-6-methyluracil: Formed through bromination.
5-Chloro-6-methyluracil: Formed through chlorination.
Scientific Research Applications
5-Iodo-6-methyluracil has several applications in scientific research:
Biology: Studied for its potential role in modifying nucleic acids and investigating DNA-protein interactions.
Medicine: Explored for its potential antiviral and anticancer properties, although more research is needed to fully understand its therapeutic potential.
Industry: Utilized in the development of novel materials and chemical sensors.
Mechanism of Action
The mechanism of action of 5-iodo-6-methyluracil involves its incorporation into nucleic acids, where it can interfere with normal base pairing and replication processes. This can lead to mutations or inhibition of DNA synthesis, making it a potential candidate for antiviral and anticancer therapies .
Comparison with Similar Compounds
- 5-Bromo-6-methyluracil
- 5-Chloro-6-methyluracil
- 6-Methyluracil
Comparison: 5-Iodo-6-methyluracil is unique due to the presence of the iodine atom, which imparts distinct chemical reactivity and biological activity compared to its brominated and chlorinated counterparts. The iodine atom’s larger size and higher electronegativity can influence the compound’s interactions with biological molecules and its overall stability .
Properties
IUPAC Name |
5-iodo-6-methyl-1H-pyrimidine-2,4-dione | |
---|---|---|
Source | PubChem | |
URL | https://pubchem.ncbi.nlm.nih.gov | |
Description | Data deposited in or computed by PubChem | |
InChI |
InChI=1S/C5H5IN2O2/c1-2-3(6)4(9)8-5(10)7-2/h1H3,(H2,7,8,9,10) | |
Source | PubChem | |
URL | https://pubchem.ncbi.nlm.nih.gov | |
Description | Data deposited in or computed by PubChem | |
InChI Key |
SYWCZCQBZPXNIV-UHFFFAOYSA-N | |
Source | PubChem | |
URL | https://pubchem.ncbi.nlm.nih.gov | |
Description | Data deposited in or computed by PubChem | |
Canonical SMILES |
CC1=C(C(=O)NC(=O)N1)I | |
Source | PubChem | |
URL | https://pubchem.ncbi.nlm.nih.gov | |
Description | Data deposited in or computed by PubChem | |
Molecular Formula |
C5H5IN2O2 | |
Source | PubChem | |
URL | https://pubchem.ncbi.nlm.nih.gov | |
Description | Data deposited in or computed by PubChem | |
DSSTOX Substance ID |
DTXSID80313064 | |
Record name | 5-Iodo-6-methyluracil | |
Source | EPA DSSTox | |
URL | https://comptox.epa.gov/dashboard/DTXSID80313064 | |
Description | DSSTox provides a high quality public chemistry resource for supporting improved predictive toxicology. | |
Molecular Weight |
252.01 g/mol | |
Source | PubChem | |
URL | https://pubchem.ncbi.nlm.nih.gov | |
Description | Data deposited in or computed by PubChem | |
CAS No. |
1461-67-2 | |
Record name | 1461-67-2 | |
Source | DTP/NCI | |
URL | https://dtp.cancer.gov/dtpstandard/servlet/dwindex?searchtype=NSC&outputformat=html&searchlist=266138 | |
Description | The NCI Development Therapeutics Program (DTP) provides services and resources to the academic and private-sector research communities worldwide to facilitate the discovery and development of new cancer therapeutic agents. | |
Explanation | Unless otherwise indicated, all text within NCI products is free of copyright and may be reused without our permission. Credit the National Cancer Institute as the source. | |
Record name | 5-Iodo-6-methyluracil | |
Source | EPA DSSTox | |
URL | https://comptox.epa.gov/dashboard/DTXSID80313064 | |
Description | DSSTox provides a high quality public chemistry resource for supporting improved predictive toxicology. | |
Record name | 5-Iodo-6-methyluracil | |
Source | European Chemicals Agency (ECHA) | |
URL | https://echa.europa.eu/information-on-chemicals | |
Description | The European Chemicals Agency (ECHA) is an agency of the European Union which is the driving force among regulatory authorities in implementing the EU's groundbreaking chemicals legislation for the benefit of human health and the environment as well as for innovation and competitiveness. | |
Explanation | Use of the information, documents and data from the ECHA website is subject to the terms and conditions of this Legal Notice, and subject to other binding limitations provided for under applicable law, the information, documents and data made available on the ECHA website may be reproduced, distributed and/or used, totally or in part, for non-commercial purposes provided that ECHA is acknowledged as the source: "Source: European Chemicals Agency, http://echa.europa.eu/". Such acknowledgement must be included in each copy of the material. ECHA permits and encourages organisations and individuals to create links to the ECHA website under the following cumulative conditions: Links can only be made to webpages that provide a link to the Legal Notice page. | |
Retrosynthesis Analysis
AI-Powered Synthesis Planning: Our tool employs the Template_relevance Pistachio, Template_relevance Bkms_metabolic, Template_relevance Pistachio_ringbreaker, Template_relevance Reaxys, Template_relevance Reaxys_biocatalysis model, leveraging a vast database of chemical reactions to predict feasible synthetic routes.
One-Step Synthesis Focus: Specifically designed for one-step synthesis, it provides concise and direct routes for your target compounds, streamlining the synthesis process.
Accurate Predictions: Utilizing the extensive PISTACHIO, BKMS_METABOLIC, PISTACHIO_RINGBREAKER, REAXYS, REAXYS_BIOCATALYSIS database, our tool offers high-accuracy predictions, reflecting the latest in chemical research and data.
Strategy Settings
Precursor scoring | Relevance Heuristic |
---|---|
Min. plausibility | 0.01 |
Model | Template_relevance |
Template Set | Pistachio/Bkms_metabolic/Pistachio_ringbreaker/Reaxys/Reaxys_biocatalysis |
Top-N result to add to graph | 6 |
Feasible Synthetic Routes
Q1: Is there any information available on the supramolecular structure of 5-iodo-6-methyluracil?
A2: The paper "The supramolecular structure of this compound" [] likely delves into this topic. Unfortunately, the abstract does not provide specific details about the structural arrangement or intermolecular interactions. Further investigation into the full text of the paper would be necessary to answer this question in detail.
Q2: Are there any analytical techniques mentioned for characterizing this compound?
A3: While not explicitly stated, the titles "OXIDATIVE HALOGENATION OF 6-METHYLURACIL" [] and "Oxidative halogenation of 6-methyluracil" [] suggest that analytical techniques commonly employed in synthetic chemistry, such as Nuclear Magnetic Resonance (NMR) spectroscopy, Infrared (IR) spectroscopy, and Mass Spectrometry (MS), were likely utilized to confirm the identity and purity of the synthesized this compound.
Disclaimer and Information on In-Vitro Research Products
Please be aware that all articles and product information presented on BenchChem are intended solely for informational purposes. The products available for purchase on BenchChem are specifically designed for in-vitro studies, which are conducted outside of living organisms. In-vitro studies, derived from the Latin term "in glass," involve experiments performed in controlled laboratory settings using cells or tissues. It is important to note that these products are not categorized as medicines or drugs, and they have not received approval from the FDA for the prevention, treatment, or cure of any medical condition, ailment, or disease. We must emphasize that any form of bodily introduction of these products into humans or animals is strictly prohibited by law. It is essential to adhere to these guidelines to ensure compliance with legal and ethical standards in research and experimentation.