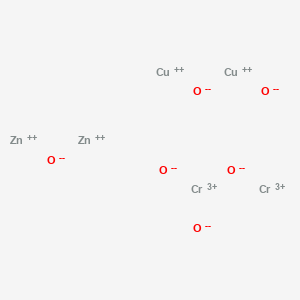
Chromium copper zinc oxide
- Click on QUICK INQUIRY to receive a quote from our team of experts.
- With the quality product at a COMPETITIVE price, you can focus more on your research.
Overview
Description
Chromium copper zinc oxide is a mixed metal oxide compound that combines the properties of chromium, copper, and zinc oxides. This compound is of significant interest due to its unique structural, electronic, and catalytic properties. It finds applications in various fields, including catalysis, electronics, and materials science.
Preparation Methods
Synthetic Routes and Reaction Conditions: Chromium copper zinc oxide can be synthesized through several methods, including co-precipitation, sol-gel, and hydrothermal synthesis. One common method is the co-precipitation technique, where aqueous solutions of chromium, copper, and zinc salts are mixed, followed by the addition of a precipitating agent such as sodium hydroxide or ammonium hydroxide. The resulting precipitate is then filtered, washed, and calcined at high temperatures (typically around 400-600°C) to obtain the desired oxide compound .
Industrial Production Methods: In industrial settings, the production of this compound often involves large-scale co-precipitation processes. The use of continuous reactors and precise control of reaction parameters, such as pH, temperature, and concentration of reactants, ensures consistent quality and high yield of the final product. Advanced techniques like spray pyrolysis and laser-based methods are also employed to produce nanostructured forms of the compound with enhanced properties .
Chemical Reactions Analysis
Types of Reactions: Chromium copper zinc oxide undergoes various chemical reactions, including oxidation, reduction, and substitution reactions. The compound’s redox properties are influenced by the presence of chromium, which can exist in multiple oxidation states (e.g., Cr(III) and Cr(VI)) .
Common Reagents and Conditions:
Oxidation: The compound can be oxidized using oxidizing agents such as hydrogen peroxide or potassium permanganate.
Reduction: Reducing agents like hydrogen gas or sodium borohydride can reduce the compound, particularly the chromium component.
Substitution: Substitution reactions can occur with halides or other ligands, leading to the formation of various coordination complexes.
Major Products: The major products formed from these reactions depend on the specific conditions and reagents used. For example, oxidation of this compound can produce higher oxidation state compounds, while reduction can yield lower oxidation state species .
Scientific Research Applications
Chromium copper zinc oxide has a wide range of scientific research applications:
Catalysis: It is used as a catalyst in various chemical reactions, including hydrogenation, dehydrogenation, and oxidation reactions. .
Materials Science: this compound is used in the development of advanced materials with specific optical, magnetic, and mechanical properties.
Environmental Applications: It is employed in the removal of pollutants from water and air, owing to its catalytic and adsorptive capabilities.
Mechanism of Action
The mechanism by which chromium copper zinc oxide exerts its effects is primarily through its redox activity and surface interactions. The compound’s ability to undergo oxidation and reduction reactions allows it to participate in various catalytic processes. The presence of chromium enhances the compound’s redox properties, while copper and zinc contribute to its structural stability and electronic characteristics .
Molecular Targets and Pathways:
Redox Reactions: Chromium ions facilitate redox reactions by cycling between different oxidation states.
Surface Interactions: The compound’s high surface area and active sites enable effective adsorption and interaction with reactants, enhancing catalytic activity.
Comparison with Similar Compounds
Chromium copper zinc oxide can be compared with other mixed metal oxides, such as:
Chromium Zinc Oxide (ZnCr2O4): This compound shares similar redox properties but lacks the additional catalytic benefits provided by copper.
Copper Zinc Oxide (CuZnO): While this compound is also used in catalysis, the presence of chromium in this compound enhances its redox activity and stability.
Chromium Copper Oxide (CuCrO2): This compound has good electrical conductivity and is used in electronic applications, but the addition of zinc in this compound improves its catalytic properties.
This compound stands out due to its unique combination of redox activity, structural stability, and catalytic efficiency, making it a versatile compound for various scientific and industrial applications.
Properties
IUPAC Name |
dicopper;dizinc;chromium(3+);oxygen(2-) |
Source
|
---|---|---|
Source | PubChem | |
URL | https://pubchem.ncbi.nlm.nih.gov | |
Description | Data deposited in or computed by PubChem | |
InChI |
InChI=1S/2Cr.2Cu.6O.2Zn/q2*+3;2*+2;6*-2;2*+2 |
Source
|
Source | PubChem | |
URL | https://pubchem.ncbi.nlm.nih.gov | |
Description | Data deposited in or computed by PubChem | |
InChI Key |
PNKGIAQRMACYGQ-UHFFFAOYSA-N |
Source
|
Source | PubChem | |
URL | https://pubchem.ncbi.nlm.nih.gov | |
Description | Data deposited in or computed by PubChem | |
Canonical SMILES |
[O-2].[O-2].[O-2].[O-2].[O-2].[O-2].[Cr+3].[Cr+3].[Cu+2].[Cu+2].[Zn+2].[Zn+2] |
Source
|
Source | PubChem | |
URL | https://pubchem.ncbi.nlm.nih.gov | |
Description | Data deposited in or computed by PubChem | |
Molecular Formula |
Cr2Cu2O6Zn2+2 |
Source
|
Source | PubChem | |
URL | https://pubchem.ncbi.nlm.nih.gov | |
Description | Data deposited in or computed by PubChem | |
Molecular Weight |
457.8 g/mol |
Source
|
Source | PubChem | |
URL | https://pubchem.ncbi.nlm.nih.gov | |
Description | Data deposited in or computed by PubChem | |
CAS No. |
1336-14-7 |
Source
|
Record name | Copper zinc chromate | |
Source | ChemIDplus | |
URL | https://pubchem.ncbi.nlm.nih.gov/substance/?source=chemidplus&sourceid=0001336147 | |
Description | ChemIDplus is a free, web search system that provides access to the structure and nomenclature authority files used for the identification of chemical substances cited in National Library of Medicine (NLM) databases, including the TOXNET system. | |
Record name | Chromium copper zinc oxide | |
Source | European Chemicals Agency (ECHA) | |
URL | https://echa.europa.eu/substance-information/-/substanceinfo/100.014.223 | |
Description | The European Chemicals Agency (ECHA) is an agency of the European Union which is the driving force among regulatory authorities in implementing the EU's groundbreaking chemicals legislation for the benefit of human health and the environment as well as for innovation and competitiveness. | |
Explanation | Use of the information, documents and data from the ECHA website is subject to the terms and conditions of this Legal Notice, and subject to other binding limitations provided for under applicable law, the information, documents and data made available on the ECHA website may be reproduced, distributed and/or used, totally or in part, for non-commercial purposes provided that ECHA is acknowledged as the source: "Source: European Chemicals Agency, http://echa.europa.eu/". Such acknowledgement must be included in each copy of the material. ECHA permits and encourages organisations and individuals to create links to the ECHA website under the following cumulative conditions: Links can only be made to webpages that provide a link to the Legal Notice page. | |
Disclaimer and Information on In-Vitro Research Products
Please be aware that all articles and product information presented on BenchChem are intended solely for informational purposes. The products available for purchase on BenchChem are specifically designed for in-vitro studies, which are conducted outside of living organisms. In-vitro studies, derived from the Latin term "in glass," involve experiments performed in controlled laboratory settings using cells or tissues. It is important to note that these products are not categorized as medicines or drugs, and they have not received approval from the FDA for the prevention, treatment, or cure of any medical condition, ailment, or disease. We must emphasize that any form of bodily introduction of these products into humans or animals is strictly prohibited by law. It is essential to adhere to these guidelines to ensure compliance with legal and ethical standards in research and experimentation.