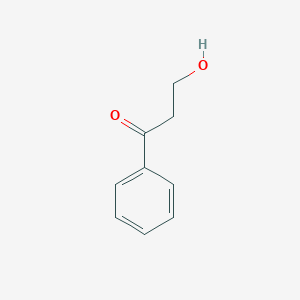
3-Hydroxy-1-phenylpropan-1-one
Overview
Description
Phenol-13C6 is a labeled isotopic compound of phenol, where all six carbon atoms in the benzene ring are replaced with the carbon-13 isotope. This compound is primarily used in scientific research to study various chemical and biological processes due to its unique isotopic labeling.
Preparation Methods
Synthetic Routes and Reaction Conditions
Phenol-13C6 can be synthesized through several methods. One common approach involves the isotopic exchange reaction where phenol is treated with carbon-13 labeled carbon dioxide under specific conditions. Another method includes the direct synthesis from benzene-13C6 through hydroxylation reactions.
Industrial Production Methods
Industrial production of Phenol-13C6 typically involves the catalytic hydroxylation of benzene-13C6. This process requires specific catalysts and reaction conditions to ensure the selective introduction of the hydroxyl group into the benzene ring.
Chemical Reactions Analysis
Types of Reactions
Phenol-13C6 undergoes various chemical reactions similar to phenol. These include:
Oxidation: Phenol-13C6 can be oxidized to quinones using oxidizing agents like potassium permanganate.
Reduction: Reduction of Phenol-13C6 can yield cyclohexanol derivatives.
Substitution: Electrophilic aromatic substitution reactions such as nitration, halogenation, and sulfonation are common.
Common Reagents and Conditions
Oxidation: Potassium permanganate in an acidic medium.
Reduction: Hydrogen gas in the presence of a palladium catalyst.
Substitution: Nitration using nitric acid and sulfuric acid, halogenation using halogens like chlorine or bromine, and sulfonation using sulfuric acid.
Major Products
Oxidation: Quinones.
Reduction: Cyclohexanol derivatives.
Substitution: Nitro, halo, and sulfonated phenol derivatives.
Scientific Research Applications
Phenol-13C6 is widely used in scientific research due to its isotopic labeling, which allows for detailed studies of chemical and biological processes. Some applications include:
Chemistry: Used in mechanistic studies of electrophilic aromatic substitution reactions.
Biology: Employed in metabolic studies to trace the incorporation and transformation of phenol in biological systems.
Medicine: Utilized in pharmacokinetic studies to understand the metabolism and distribution of phenol-based drugs.
Industry: Applied in the development of new materials and chemicals through isotopic labeling techniques.
Mechanism of Action
Phenol-13C6 exerts its effects through similar mechanisms as phenol. It acts as a potent proteolytic agent, dissolving tissues on contact via proteolysis. In high concentrations, it can produce chemical neurolysis when injected next to a nerve, affecting nerve fibers nonselectively .
Comparison with Similar Compounds
Phenol-13C6 can be compared with other isotopically labeled phenols and related compounds:
Pentachlorophenol-13C6: A chlorinated derivative used in environmental studies.
Salicylic acid (phenyl-13C6): Used in pharmaceutical research.
Benzene-13C6: A precursor for various labeled aromatic compounds.
Phenol-13C6 is unique due to its specific isotopic labeling, which makes it highly valuable for detailed mechanistic and metabolic studies.
Properties
IUPAC Name |
3-hydroxy-1-phenylpropan-1-one | |
---|---|---|
Source | PubChem | |
URL | https://pubchem.ncbi.nlm.nih.gov | |
Description | Data deposited in or computed by PubChem | |
InChI |
InChI=1S/C9H10O2/c10-7-6-9(11)8-4-2-1-3-5-8/h1-5,10H,6-7H2 | |
Source | PubChem | |
URL | https://pubchem.ncbi.nlm.nih.gov | |
Description | Data deposited in or computed by PubChem | |
InChI Key |
PQCFUZMQHVIOSM-UHFFFAOYSA-N | |
Source | PubChem | |
URL | https://pubchem.ncbi.nlm.nih.gov | |
Description | Data deposited in or computed by PubChem | |
Canonical SMILES |
C1=CC=C(C=C1)C(=O)CCO | |
Source | PubChem | |
URL | https://pubchem.ncbi.nlm.nih.gov | |
Description | Data deposited in or computed by PubChem | |
Molecular Formula |
C9H10O2 | |
Source | PubChem | |
URL | https://pubchem.ncbi.nlm.nih.gov | |
Description | Data deposited in or computed by PubChem | |
DSSTOX Substance ID |
DTXSID10927537 | |
Record name | 3-Hydroxy-1-phenylpropan-1-one | |
Source | EPA DSSTox | |
URL | https://comptox.epa.gov/dashboard/DTXSID10927537 | |
Description | DSSTox provides a high quality public chemistry resource for supporting improved predictive toxicology. | |
Molecular Weight |
150.17 g/mol | |
Source | PubChem | |
URL | https://pubchem.ncbi.nlm.nih.gov | |
Description | Data deposited in or computed by PubChem | |
CAS No. |
1321-48-8, 5650-41-9 | |
Record name | Hydroxypropiophenone | |
Source | ChemIDplus | |
URL | https://pubchem.ncbi.nlm.nih.gov/substance/?source=chemidplus&sourceid=0001321488 | |
Description | ChemIDplus is a free, web search system that provides access to the structure and nomenclature authority files used for the identification of chemical substances cited in National Library of Medicine (NLM) databases, including the TOXNET system. | |
Record name | 3-Hydroxypropiophenone | |
Source | ChemIDplus | |
URL | https://pubchem.ncbi.nlm.nih.gov/substance/?source=chemidplus&sourceid=0005650419 | |
Description | ChemIDplus is a free, web search system that provides access to the structure and nomenclature authority files used for the identification of chemical substances cited in National Library of Medicine (NLM) databases, including the TOXNET system. | |
Record name | 3-Hydroxy-1-phenylpropan-1-one | |
Source | EPA DSSTox | |
URL | https://comptox.epa.gov/dashboard/DTXSID10927537 | |
Description | DSSTox provides a high quality public chemistry resource for supporting improved predictive toxicology. | |
Record name | 3-HYDROXYPROPIOPHENONE | |
Source | FDA Global Substance Registration System (GSRS) | |
URL | https://gsrs.ncats.nih.gov/ginas/app/beta/substances/V3OOP8T819 | |
Description | The FDA Global Substance Registration System (GSRS) enables the efficient and accurate exchange of information on what substances are in regulated products. Instead of relying on names, which vary across regulatory domains, countries, and regions, the GSRS knowledge base makes it possible for substances to be defined by standardized, scientific descriptions. | |
Explanation | Unless otherwise noted, the contents of the FDA website (www.fda.gov), both text and graphics, are not copyrighted. They are in the public domain and may be republished, reprinted and otherwise used freely by anyone without the need to obtain permission from FDA. Credit to the U.S. Food and Drug Administration as the source is appreciated but not required. | |
Synthesis routes and methods
Procedure details
Disclaimer and Information on In-Vitro Research Products
Please be aware that all articles and product information presented on BenchChem are intended solely for informational purposes. The products available for purchase on BenchChem are specifically designed for in-vitro studies, which are conducted outside of living organisms. In-vitro studies, derived from the Latin term "in glass," involve experiments performed in controlled laboratory settings using cells or tissues. It is important to note that these products are not categorized as medicines or drugs, and they have not received approval from the FDA for the prevention, treatment, or cure of any medical condition, ailment, or disease. We must emphasize that any form of bodily introduction of these products into humans or animals is strictly prohibited by law. It is essential to adhere to these guidelines to ensure compliance with legal and ethical standards in research and experimentation.