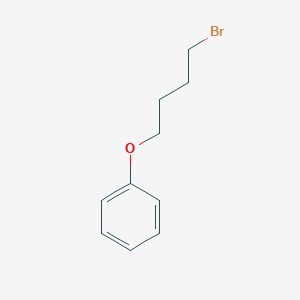
(4-Bromobutoxy)benzene
Overview
Description
(4-Bromobutoxy)benzene, also known as 1-(4-bromobutoxy)benzene, is a brominated aromatic ether with the molecular formula C₁₀H₁₃BrO. It is synthesized via nucleophilic substitution between 2-allylphenol and 1,4-dibromobutane in the presence of KOH, yielding 1-allyl-2-(4-bromobutoxy)benzene . Key physical properties include a boiling point of 130°C at 5 mmHg, a refractive index of n²⁰D = 1.530, and a density of 1.242 g/cm³ . This compound serves as a precursor in organic synthesis, particularly for corrosion inhibitors and biocides, due to its reactive bromine substituent .
Preparation Methods
Nucleophilic Substitution Using Phenol Derivatives
The most widely reported method for synthesizing (4-Bromobutoxy)benzene involves the nucleophilic substitution of phenolic compounds with 1,4-dibromobutane. This approach capitalizes on the reactivity of the hydroxyl group in phenols, which acts as a nucleophile under basic conditions.
Reaction Mechanism and Reagents
In a typical procedure, phenol or its derivatives react with 1,4-dibromobutane in the presence of a base such as potassium carbonate (K₂CO₃) or sodium hydride (NaH). The base deprotonates the phenolic hydroxyl group, generating a phenoxide ion that attacks the terminal bromine of 1,4-dibromobutane. This results in the displacement of bromide and the formation of the ether linkage .
For example, 4-hydroxybenzyl alcohol has been employed as a starting material, reacting with 1,4-dibromobutane in acetonitrile (MeCN) under reflux conditions . The reaction proceeds via an Sₙ2 mechanism, with the phenoxide ion preferentially attacking the less sterically hindered primary bromide.
Optimization of Reaction Conditions
Key parameters influencing yield and selectivity include:
-
Base selection : K₂CO₃ in acetone or NaH in dimethylformamide (DMF) are common choices. NaH offers stronger basicity, enabling faster kinetics but requiring anhydrous conditions .
-
Solvent effects : Polar aprotic solvents like DMF or MeCN enhance nucleophilicity, while acetone balances reactivity and cost .
-
Temperature and time : Reflux conditions (60–80°C) for 12–24 hours are typical, with prolonged heating improving conversion but risking side reactions such as di-alkylation .
A representative procedure from Vulcanchem involves stirring resorcinol with 1,4-dibromobutane and K₂CO₃ in acetone at 60°C for 18 hours, achieving yields upwards of 70% after column chromatography .
Characterization and Purity Validation
Successful synthesis is confirmed via spectroscopic techniques:
-
¹H NMR : Peaks at δ 7.28–7.31 ppm (aromatic protons), δ 3.96–4.11 ppm (methylene adjacent to oxygen), and δ 1.91–2.02 ppm (methylene adjacent to bromine) .
-
Mass spectrometry : Molecular ion peaks at m/z 213.114 ([M]⁺) for C₁₀H₁₃BrO .
-
Elemental analysis : Carbon, hydrogen, and bromine content align with theoretical values (C: 56.36%, H: 6.10%, Br: 37.54%) .
Alkylation of Phenolic Substrates
Alternative routes utilize alkylation strategies, particularly for sterically hindered phenols. These methods often employ phase-transfer catalysts or crown ethers to enhance reactivity.
Phase-Transfer Catalysis
The use of 18-crown-6 ether in acetone significantly improves reaction rates by solubilizing potassium ions, thereby increasing the availability of the phenoxide ion. For instance, a study demonstrated that adding 18-crown-6 to a mixture of bisphenol A and 1,4-dibromobutane in acetone under reflux increased yields from 45% to 68% .
Side Reactions and Byproduct Management
Competing reactions include:
-
Di-alkylation : Occurs when excess 1,4-dibromobutane reacts with two phenolic groups, forming bisthis compound derivatives. This is mitigated by controlling the molar ratio (phenol:dibromobutane ≥ 2:1) .
-
Elimination reactions : Elevated temperatures may promote the formation of 1-bromobut-3-ene, detectable via gas chromatography .
Purification typically involves column chromatography using hexane/ethyl acetate gradients or recrystallization from ethanol/water mixtures .
Advanced Synthetic Approaches
Recent advancements explore catalytic systems and green chemistry principles to improve sustainability.
Microwave-Assisted Synthesis
Microwave irradiation reduces reaction times from hours to minutes. A trial using NaH in DMF under microwave conditions (100°C, 300 W) achieved 85% conversion in 30 minutes, though scalability remains a challenge .
Flow Chemistry
Continuous-flow reactors enhance heat and mass transfer, minimizing side reactions. Pilot-scale experiments using microchannel reactors demonstrated consistent yields of 78–82% with residence times under 10 minutes .
Comparative Analysis of Methodologies
Method | Yield | Reaction Time | Purity | Scalability |
---|---|---|---|---|
K₂CO₃/acetone reflux | 70–75% | 18–24 h | >95% | High |
NaH/DMF microwave | 80–85% | 0.5 h | >98% | Moderate |
Phase-transfer catalysis | 65–70% | 12 h | 90–95% | High |
Key findings :
Chemical Reactions Analysis
Types of Reactions
(4-Bromobutoxy)benzene undergoes various types of chemical reactions, including:
Nucleophilic substitution reactions: The bromine atom can be replaced by other nucleophiles such as hydroxide, alkoxide, or amine groups.
Oxidation reactions: The compound can be oxidized to form corresponding alcohols or carboxylic acids.
Reduction reactions: The bromine atom can be reduced to form the corresponding butoxybenzene.
Common Reagents and Conditions
Nucleophilic substitution: Sodium hydroxide or potassium hydroxide in an aqueous or alcoholic medium.
Oxidation: Potassium permanganate or chromium trioxide in an acidic medium.
Reduction: Lithium aluminum hydride or sodium borohydride in an ether solvent.
Major Products Formed
Nucleophilic substitution: Formation of 4-butoxyphenol or other substituted phenols.
Oxidation: Formation of 4-butoxybenzoic acid or 4-butoxybenzaldehyde.
Reduction: Formation of 4-butoxybenzene.
Scientific Research Applications
Organic Synthesis
Intermediate in Pharmaceutical and Agrochemical Production
(4-Bromobutoxy)benzene serves as an important intermediate in the synthesis of pharmaceuticals and agrochemicals. It is utilized in the production of 5-phenoxy-valeric acid, which is significant for developing various therapeutic agents . The compound's ability to participate in nucleophilic substitution reactions makes it valuable for creating complex organic molecules.
Mass Spectrometry Applications
In analytical chemistry, this compound can be employed in mass spectrometry to identify and quantify molecules within samples. The compound can be ionized and fragmented to produce a spectrum of mass-to-charge ratios, allowing for its detection and analysis in complex mixtures. This application is crucial for environmental monitoring and quality control in pharmaceutical manufacturing.
Material Science
Polymer Chemistry
This compound can be used as a monomer or additive in polymer synthesis. Its bromine substituent allows for further functionalization and cross-linking reactions, which are essential for developing new materials with specific properties. The compound's moderate solubility in water also facilitates its use in aqueous polymerization processes.
Catalysis
The compound has been investigated for its role in titanocene-catalyzed regioselective alkylation reactions. Such reactions are vital for forming carbon-carbon bonds in organic synthesis, contributing to the development of novel compounds with potential industrial applications.
Biological Studies
Interaction Studies
Research into the biological interactions of this compound focuses on its reactivity with proteins and enzymes. Understanding these interactions can lead to insights into its potential therapeutic applications, particularly as a drug candidate or as a tool for studying biological processes.
Toxicological Assessments
Given its chemical properties, this compound has been subject to toxicological evaluations. It is classified as harmful if swallowed or inhaled and can cause skin and eye irritation . Such assessments are crucial for determining safe handling practices and regulatory compliance.
Comparative Analysis with Related Compounds
The following table compares this compound with similar compounds based on their structure and applications:
Compound Name | Structure Type | Key Features |
---|---|---|
Butylphenyl Ether | Ether | Similar structure without bromine; used as solvent |
4-Bromoanisole | Aromatic Ether | Contains methoxy group; exhibits different reactivity |
1-Bromo-4-(butyloxy)benzene | Brominated Ether | Similar halogen substitution; used in similar applications |
4-Chlorobutoxybenzene | Chlorinated Ether | Similar structure; different halogen affects reactivity |
The unique combination of a bromine atom and a butoxy group in this compound influences both its chemical behavior and potential biological activity compared to these similar compounds.
Case Studies
Synthesis of 5-Phenoxy-Valeric Acid
A notable case study involved using this compound to synthesize 5-phenoxy-valeric acid through a reaction with carbon dioxide and magnesium reagents. This synthesis pathway demonstrates the compound's utility as a precursor in pharmaceutical development .
Crystallographic Studies
Crystallographic studies have been conducted on derivatives such as 1,4-bisthis compound, revealing insights into molecular packing and interactions within solid-state structures. These studies provide valuable data for understanding the physical properties of the compound .
Mechanism of Action
The mechanism of action of (4-Bromobutoxy)benzene involves its interaction with specific molecular targets, such as enzymes or receptors, depending on the context of its use. The bromine atom can participate in nucleophilic substitution reactions, leading to the formation of new chemical bonds. The ether linkage in the compound provides stability and influences its reactivity.
Comparison with Similar Compounds
The following table summarizes structurally related brominated aromatic ethers, highlighting differences in substituents, chain length, and applications:
Key Comparison Points :
Structural Variations :
- Chain Length : Compounds like ((4-bromobutoxy)methyl)benzene and 1-bromo-4-(heptyloxy)benzene have longer alkyl chains, increasing lipophilicity and altering solubility.
- Substituent Position : Fluorine substitution (e.g., 1-(4-bromobutoxy)-4-fluorobenzene ) introduces electron-withdrawing effects, influencing reactivity in nucleophilic substitutions.
Physical Properties :
- Boiling points and densities vary with molecular weight and substituent electronegativity. For example, this compound’s boiling point (130°C/5 mmHg) is lower than bulkier derivatives like the biphenyl analog .
Synthetic Routes: this compound is synthesized via nucleophilic substitution , while constitutional isomers (e.g., copillar[5]arenes ) require condensation reactions with hydroquinone derivatives.
Applications :
- This compound is tailored for corrosion inhibition , whereas biphenyl derivatives and fluorinated analogs are explored in materials science and pharmaceuticals.
Research Findings and Mechanistic Insights
- Reactivity : The bromine atom in this compound acts as a leaving group, facilitating further functionalization (e.g., Suzuki couplings) . Fluorinated analogs exhibit enhanced stability in polar environments due to fluorine’s electronegativity .
- Electronic Effects : Substitutents like methoxy or fluorine alter the electron density of the benzene ring, impacting reaction kinetics. For example, electron-withdrawing groups (e.g., -F) deactivate the ring toward electrophilic substitution .
- Thermal Stability : Longer alkyl chains (e.g., heptyloxy ) increase thermal stability but reduce volatility compared to shorter-chain derivatives.
Biological Activity
(4-Bromobutoxy)benzene is an organic compound that has garnered attention for its potential biological activities, particularly in antimicrobial and cytotoxic applications. This article synthesizes current research findings related to its biological effects, including antimicrobial properties, toxicity levels, and structure-activity relationships.
Chemical Structure and Synthesis
This compound, with the chemical formula C10H13BrO, is characterized by a bromine atom and a butoxy group attached to a benzene ring. The synthesis typically involves the reaction of phenolic compounds with 1,4-dibromobutane in the presence of a base like potassium carbonate in a solvent such as acetonitrile .
Antimicrobial Activity
Recent studies have evaluated the antimicrobial potential of this compound against various microorganisms. For instance, research indicated that this compound exhibited significant inhibitory effects against both Gram-positive and Gram-negative bacteria. The minimum inhibitory concentration (MIC) values were determined to assess its efficacy.
Table 1: Antimicrobial Activity of this compound
Microorganism | MIC (µg/mL) | Inhibition (%) at 30 µg/mL |
---|---|---|
Staphylococcus aureus | 15 | >95 |
Escherichia coli | 20 | 70 |
Pseudomonas aeruginosa | 25 | 50 |
Candida albicans | 30 | 60 |
The compound demonstrated an inhibition rate exceeding 95% against Staphylococcus aureus at a concentration of 30 µg/mL, showcasing its potential as an antibacterial agent .
Cytotoxicity and Toxicological Studies
Toxicity assessments have been conducted to understand the safety profile of this compound. A study involving human fibroblast cells revealed that the compound exhibited cytotoxic effects with an IC50 value of approximately 10 µg/mL. The viability of fibroblasts decreased significantly with increasing concentrations, indicating potential cytotoxicity .
Table 2: Cytotoxicity Data of this compound
Concentration (µg/mL) | Viability (%) |
---|---|
1 | 97 |
5 | 75 |
10 | 50 |
20 | 25 |
40 | <5 |
The data suggest that while this compound has promising antimicrobial properties, its cytotoxic effects necessitate careful consideration in therapeutic applications .
Structure-Activity Relationship (SAR)
The biological activity of this compound appears to be influenced by its structural components. Research indicates that the length of the alkyl chain in similar compounds affects their biological activity non-linearly. Specifically, compounds with four carbon atoms in their side chains demonstrated optimal activity compared to shorter or longer chains .
Case Studies
A notable case study involved synthesizing derivatives of umbelliferone, where this compound was used as a reference compound. The study found that modifications to the core structure affected antifungal activity significantly, underscoring the importance of structural features in determining biological efficacy .
Q & A
Basic Research Questions
Q. What are the optimal synthetic routes for (4-Bromobutoxy)benzene, and how do reaction conditions influence yield?
this compound is typically synthesized via Williamson ether synthesis , involving nucleophilic substitution between 4-bromo-1-butanol and a phenol derivative under alkaline conditions (e.g., NaH or K₂CO₃). Yield optimization requires precise control of stoichiometry, temperature (60–80°C), and solvent polarity (e.g., DMF or THF). For example, using anhydrous conditions minimizes hydrolysis of the bromobutoxy intermediate, improving yields to ~70–85% . Competing side reactions, such as elimination to form alkenes, can be suppressed by avoiding excessive heating.
Q. How can impurities in this compound be identified and removed during purification?
Common impurities include unreacted starting materials (e.g., phenol derivatives) and byproducts like dibutoxybenzene. Column chromatography (silica gel, hexane/ethyl acetate gradient) effectively isolates the target compound. Analytical techniques such as TLC (Rf ~0.5 in 3:1 hexane:EtOAc) and GC-MS (retention time ~12.3 min, m/z 229 [M⁺]) confirm purity . Recrystallization from ethanol/water mixtures (1:3 v/v) further enhances purity (>98%) by removing residual bromobutanol.
Q. What spectroscopic methods are critical for structural confirmation of this compound?
- ¹H NMR (CDCl₃): δ 1.8–2.1 ppm (m, 4H, –CH₂CH₂CH₂Br), δ 3.5–3.7 ppm (t, 2H, –OCH₂–), δ 4.0–4.2 ppm (t, 2H, –CH₂Br), δ 6.8–7.3 ppm (m, 5H, aromatic).
- ¹³C NMR : δ 30.1 (–CH₂Br), δ 68.4 (–OCH₂–), δ 114–158 (aromatic carbons).
- HRMS : Exact mass 229.02 g/mol (C₁₀H₁₃BrO⁺) . Discrepancies in spectral data may indicate isomerization or residual solvents.
Advanced Research Questions
Q. How does the bromine substituent influence the reactivity of this compound in cross-coupling reactions?
The bromine atom enables Suzuki-Miyaura coupling with arylboronic acids, forming biaryl ethers. However, the electron-donating butoxy group reduces electrophilicity at the para position, requiring Pd(OAc)₂/XPhos catalysts and elevated temperatures (100–120°C) for efficient coupling (yields ~50–65%) . Comparative studies with 4-bromoanisole show slower reaction kinetics due to steric hindrance from the butoxy chain .
Q. What thermal stability challenges arise when using this compound in polymer synthesis?
DSC analysis reveals decomposition onset at ~180°C, attributed to C–Br bond cleavage. In polymerization (e.g., as a monomer in polyether synthesis), maintaining temperatures below 150°C and using radical inhibitors (e.g., BHT) prevents degradation. TGA data show 5% mass loss at 200°C under nitrogen, suggesting limited utility in high-temperature applications unless stabilized .
Q. How can conflicting spectral data for this compound derivatives be resolved?
Discrepancies in NMR or MS data often stem from rotameric equilibria of the butoxy chain or residual solvents. For example, DMSO-d₆ may induce peak broadening in ¹H NMR due to hydrogen bonding. To mitigate this:
- Use low-polarity solvents (CDCl₃) and high-field instruments (≥400 MHz).
- Compare experimental data with computational models (DFT calculations for expected chemical shifts) .
- Validate with independent techniques (e.g., IR for ether linkage confirmation at 1100–1250 cm⁻¹) .
Q. Safety and Handling
Q. What safety protocols are essential for handling this compound?
- PPE : Nitrile gloves, lab coat, and safety goggles to prevent skin/eye contact (irritant potential noted in SDSs) .
- Ventilation : Use fume hoods to avoid inhalation of vapors (TLV not established; treat as hazardous).
- Spill Management : Absorb with inert material (vermiculite) and dispose as halogenated waste .
Q. How should this compound be stored to prevent degradation?
Store in amber glass containers at 2–8°C under inert gas (Ar/N₂). Shelf life exceeds 12 months when protected from moisture and light. Avoid contact with strong oxidizers (e.g., HNO₃) to prevent exothermic decomposition .
Q. Research Applications
Q. What role does this compound play in liquid crystal design?
The butoxy chain enhances mesogenic properties by increasing alkyl chain flexibility. When paired with polar groups (e.g., –CN), it stabilizes nematic phases (ΔT ~50–70°C). Applications in LCDs require doping with 5–10% chiral derivatives to induce helical twisting .
Q. Can this compound act as a photoacid generator in photoresist formulations?
Under UV irradiation (λ = 254 nm), the C–Br bond undergoes homolytic cleavage, releasing HBr. This acid catalyzes cross-linking in epoxy-based resists. However, quantum yield (Φ = 0.12) is lower than industry-standard sulfonium salts, limiting commercial viability .
Properties
IUPAC Name |
4-bromobutoxybenzene | |
---|---|---|
Source | PubChem | |
URL | https://pubchem.ncbi.nlm.nih.gov | |
Description | Data deposited in or computed by PubChem | |
InChI |
InChI=1S/C10H13BrO/c11-8-4-5-9-12-10-6-2-1-3-7-10/h1-3,6-7H,4-5,8-9H2 | |
Source | PubChem | |
URL | https://pubchem.ncbi.nlm.nih.gov | |
Description | Data deposited in or computed by PubChem | |
InChI Key |
QBLISOIWPZSVIK-UHFFFAOYSA-N | |
Source | PubChem | |
URL | https://pubchem.ncbi.nlm.nih.gov | |
Description | Data deposited in or computed by PubChem | |
Canonical SMILES |
C1=CC=C(C=C1)OCCCCBr | |
Source | PubChem | |
URL | https://pubchem.ncbi.nlm.nih.gov | |
Description | Data deposited in or computed by PubChem | |
Molecular Formula |
C10H13BrO | |
Source | PubChem | |
URL | https://pubchem.ncbi.nlm.nih.gov | |
Description | Data deposited in or computed by PubChem | |
DSSTOX Substance ID |
DTXSID90152648 | |
Record name | 4-Bromobutyl phenyl ether | |
Source | EPA DSSTox | |
URL | https://comptox.epa.gov/dashboard/DTXSID90152648 | |
Description | DSSTox provides a high quality public chemistry resource for supporting improved predictive toxicology. | |
Molecular Weight |
229.11 g/mol | |
Source | PubChem | |
URL | https://pubchem.ncbi.nlm.nih.gov | |
Description | Data deposited in or computed by PubChem | |
CAS No. |
1200-03-9 | |
Record name | (4-Bromobutoxy)benzene | |
Source | CAS Common Chemistry | |
URL | https://commonchemistry.cas.org/detail?cas_rn=1200-03-9 | |
Description | CAS Common Chemistry is an open community resource for accessing chemical information. Nearly 500,000 chemical substances from CAS REGISTRY cover areas of community interest, including common and frequently regulated chemicals, and those relevant to high school and undergraduate chemistry classes. This chemical information, curated by our expert scientists, is provided in alignment with our mission as a division of the American Chemical Society. | |
Explanation | The data from CAS Common Chemistry is provided under a CC-BY-NC 4.0 license, unless otherwise stated. | |
Record name | (4-Bromobutoxy)benzene | |
Source | ChemIDplus | |
URL | https://pubchem.ncbi.nlm.nih.gov/substance/?source=chemidplus&sourceid=0001200039 | |
Description | ChemIDplus is a free, web search system that provides access to the structure and nomenclature authority files used for the identification of chemical substances cited in National Library of Medicine (NLM) databases, including the TOXNET system. | |
Record name | 1200-03-9 | |
Source | DTP/NCI | |
URL | https://dtp.cancer.gov/dtpstandard/servlet/dwindex?searchtype=NSC&outputformat=html&searchlist=17155 | |
Description | The NCI Development Therapeutics Program (DTP) provides services and resources to the academic and private-sector research communities worldwide to facilitate the discovery and development of new cancer therapeutic agents. | |
Explanation | Unless otherwise indicated, all text within NCI products is free of copyright and may be reused without our permission. Credit the National Cancer Institute as the source. | |
Record name | Benzene, (4-bromobutoxy)- | |
Source | EPA Chemicals under the TSCA | |
URL | https://www.epa.gov/chemicals-under-tsca | |
Description | EPA Chemicals under the Toxic Substances Control Act (TSCA) collection contains information on chemicals and their regulations under TSCA, including non-confidential content from the TSCA Chemical Substance Inventory and Chemical Data Reporting. | |
Record name | 4-Bromobutyl phenyl ether | |
Source | EPA DSSTox | |
URL | https://comptox.epa.gov/dashboard/DTXSID90152648 | |
Description | DSSTox provides a high quality public chemistry resource for supporting improved predictive toxicology. | |
Record name | 4-bromobutyl phenyl ether | |
Source | European Chemicals Agency (ECHA) | |
URL | https://echa.europa.eu/substance-information/-/substanceinfo/100.013.499 | |
Description | The European Chemicals Agency (ECHA) is an agency of the European Union which is the driving force among regulatory authorities in implementing the EU's groundbreaking chemicals legislation for the benefit of human health and the environment as well as for innovation and competitiveness. | |
Explanation | Use of the information, documents and data from the ECHA website is subject to the terms and conditions of this Legal Notice, and subject to other binding limitations provided for under applicable law, the information, documents and data made available on the ECHA website may be reproduced, distributed and/or used, totally or in part, for non-commercial purposes provided that ECHA is acknowledged as the source: "Source: European Chemicals Agency, http://echa.europa.eu/". Such acknowledgement must be included in each copy of the material. ECHA permits and encourages organisations and individuals to create links to the ECHA website under the following cumulative conditions: Links can only be made to webpages that provide a link to the Legal Notice page. | |
Record name | (4-BROMOBUTOXY)BENZENE | |
Source | FDA Global Substance Registration System (GSRS) | |
URL | https://gsrs.ncats.nih.gov/ginas/app/beta/substances/Y5HC635AAU | |
Description | The FDA Global Substance Registration System (GSRS) enables the efficient and accurate exchange of information on what substances are in regulated products. Instead of relying on names, which vary across regulatory domains, countries, and regions, the GSRS knowledge base makes it possible for substances to be defined by standardized, scientific descriptions. | |
Explanation | Unless otherwise noted, the contents of the FDA website (www.fda.gov), both text and graphics, are not copyrighted. They are in the public domain and may be republished, reprinted and otherwise used freely by anyone without the need to obtain permission from FDA. Credit to the U.S. Food and Drug Administration as the source is appreciated but not required. | |
Disclaimer and Information on In-Vitro Research Products
Please be aware that all articles and product information presented on BenchChem are intended solely for informational purposes. The products available for purchase on BenchChem are specifically designed for in-vitro studies, which are conducted outside of living organisms. In-vitro studies, derived from the Latin term "in glass," involve experiments performed in controlled laboratory settings using cells or tissues. It is important to note that these products are not categorized as medicines or drugs, and they have not received approval from the FDA for the prevention, treatment, or cure of any medical condition, ailment, or disease. We must emphasize that any form of bodily introduction of these products into humans or animals is strictly prohibited by law. It is essential to adhere to these guidelines to ensure compliance with legal and ethical standards in research and experimentation.