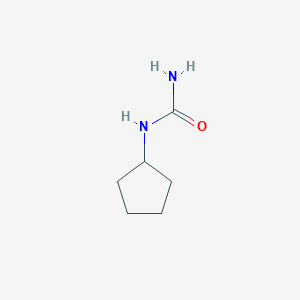
Cyclopentylurea
Overview
Description
Cyclopentylurea is a useful research compound. Its molecular formula is C6H12N2O and its molecular weight is 128.17 g/mol. The purity is usually 95%.
The exact mass of the compound this compound is unknown and the complexity rating of the compound is unknown. The compound has been submitted to the National Cancer Institute (NCI) for testing and evaluation and the Cancer Chemotherapy National Service Center (NSC) number is 163150. The United Nations designated GHS hazard class pictogram is Irritant, and the GHS signal word is WarningThe storage condition is unknown. Please store according to label instructions upon receipt of goods.
BenchChem offers high-quality this compound suitable for many research applications. Different packaging options are available to accommodate customers' requirements. Please inquire for more information about this compound including the price, delivery time, and more detailed information at info@benchchem.com.
Scientific Research Applications
Cyclodextrins and Derivatives : Cyclopentylurea is structurally different from cyclodextrins, but both involve cyclic structures. Cyclodextrins are cyclic oligosaccharides with applications in pharmaceuticals, drug delivery, cosmetics, and the food industry. They form host-guest type inclusion complexes, modifying physical and chemical properties of molecules, which could be a principle relevant to this compound's potential applications (Sharma & Baldi, 2016).
Drug Metabolism and Enzyme Interaction : Understanding the interaction of compounds with enzymes is crucial in drug discovery and development. This compound, like other small molecules, could potentially be a substrate or inhibitor of enzymes such as cytochrome P450s. This enzyme group metabolizes over 100 clinically used drugs, indicating the significance of studying such interactions for any new compound (He et al., 2011).
Cyclophosphamide and Related Compounds : Cyclophosphamide, a chemotherapeutic and immunosuppressant drug, is an example of a cyclo-compound with significant medical applications. Studying its mechanisms and effects could provide insights into the potential pathways and effects of this compound in biological systems (Tashkin et al., 2007).
Cyclotides and Protein Engineering : Cyclotides are stable plant proteins with various bioactivities and potential in drug design. They demonstrate the utility of cyclic structures in biological applications, which might be relevant to the structural analysis and application of this compound (Henriques & Craik, 2010).
Impact on Biological Systems : Studies on drugs like cyclosporine provide insights into the potential effects of other cyclo-compounds, including this compound, on biological systems, such as neuronal and glial cells (McDonald et al., 1996).
Mechanism of Action
Biochemical Analysis
Biochemical Properties
Cyclopentylurea interacts with the NS3 protease, a critical enzyme in the life cycle of the hepatitis C virus . By binding to the active site of this enzyme, this compound blocks its activity, thereby inhibiting the replication of the virus .
Cellular Effects
The primary cellular effect of this compound is the inhibition of the NS3 protease . This enzyme is essential for the replication of the hepatitis C virus, so its inhibition by this compound can effectively halt the spread of the virus within the cell .
Molecular Mechanism
This compound exerts its effects at the molecular level by binding to the active site of the NS3 protease . This binding interaction inhibits the enzyme’s activity, preventing it from participating in the replication of the hepatitis C virus .
Metabolic Pathways
Given its role as an inhibitor of the NS3 protease, it is likely that it interacts with enzymes and cofactors involved in the replication of the hepatitis C virus .
Properties
IUPAC Name |
cyclopentylurea | |
---|---|---|
Source | PubChem | |
URL | https://pubchem.ncbi.nlm.nih.gov | |
Description | Data deposited in or computed by PubChem | |
InChI |
InChI=1S/C6H12N2O/c7-6(9)8-5-3-1-2-4-5/h5H,1-4H2,(H3,7,8,9) | |
Source | PubChem | |
URL | https://pubchem.ncbi.nlm.nih.gov | |
Description | Data deposited in or computed by PubChem | |
InChI Key |
CBEYJGNJOCTQGW-UHFFFAOYSA-N | |
Source | PubChem | |
URL | https://pubchem.ncbi.nlm.nih.gov | |
Description | Data deposited in or computed by PubChem | |
Canonical SMILES |
C1CCC(C1)NC(=O)N | |
Source | PubChem | |
URL | https://pubchem.ncbi.nlm.nih.gov | |
Description | Data deposited in or computed by PubChem | |
Molecular Formula |
C6H12N2O | |
Source | PubChem | |
URL | https://pubchem.ncbi.nlm.nih.gov | |
Description | Data deposited in or computed by PubChem | |
DSSTOX Substance ID |
DTXSID90303875 | |
Record name | cyclopentyl-urea | |
Source | EPA DSSTox | |
URL | https://comptox.epa.gov/dashboard/DTXSID90303875 | |
Description | DSSTox provides a high quality public chemistry resource for supporting improved predictive toxicology. | |
Molecular Weight |
128.17 g/mol | |
Source | PubChem | |
URL | https://pubchem.ncbi.nlm.nih.gov | |
Description | Data deposited in or computed by PubChem | |
CAS No. |
1194-06-5 | |
Record name | 1194-06-5 | |
Source | DTP/NCI | |
URL | https://dtp.cancer.gov/dtpstandard/servlet/dwindex?searchtype=NSC&outputformat=html&searchlist=163150 | |
Description | The NCI Development Therapeutics Program (DTP) provides services and resources to the academic and private-sector research communities worldwide to facilitate the discovery and development of new cancer therapeutic agents. | |
Explanation | Unless otherwise indicated, all text within NCI products is free of copyright and may be reused without our permission. Credit the National Cancer Institute as the source. | |
Record name | cyclopentyl-urea | |
Source | EPA DSSTox | |
URL | https://comptox.epa.gov/dashboard/DTXSID90303875 | |
Description | DSSTox provides a high quality public chemistry resource for supporting improved predictive toxicology. | |
Record name | CYCLOPENTYLUREA | |
Source | European Chemicals Agency (ECHA) | |
URL | https://echa.europa.eu/information-on-chemicals | |
Description | The European Chemicals Agency (ECHA) is an agency of the European Union which is the driving force among regulatory authorities in implementing the EU's groundbreaking chemicals legislation for the benefit of human health and the environment as well as for innovation and competitiveness. | |
Explanation | Use of the information, documents and data from the ECHA website is subject to the terms and conditions of this Legal Notice, and subject to other binding limitations provided for under applicable law, the information, documents and data made available on the ECHA website may be reproduced, distributed and/or used, totally or in part, for non-commercial purposes provided that ECHA is acknowledged as the source: "Source: European Chemicals Agency, http://echa.europa.eu/". Such acknowledgement must be included in each copy of the material. ECHA permits and encourages organisations and individuals to create links to the ECHA website under the following cumulative conditions: Links can only be made to webpages that provide a link to the Legal Notice page. | |
Synthesis routes and methods I
Procedure details
Synthesis routes and methods II
Procedure details
Synthesis routes and methods III
Procedure details
Synthesis routes and methods IV
Procedure details
Synthesis routes and methods V
Procedure details
Retrosynthesis Analysis
AI-Powered Synthesis Planning: Our tool employs the Template_relevance Pistachio, Template_relevance Bkms_metabolic, Template_relevance Pistachio_ringbreaker, Template_relevance Reaxys, Template_relevance Reaxys_biocatalysis model, leveraging a vast database of chemical reactions to predict feasible synthetic routes.
One-Step Synthesis Focus: Specifically designed for one-step synthesis, it provides concise and direct routes for your target compounds, streamlining the synthesis process.
Accurate Predictions: Utilizing the extensive PISTACHIO, BKMS_METABOLIC, PISTACHIO_RINGBREAKER, REAXYS, REAXYS_BIOCATALYSIS database, our tool offers high-accuracy predictions, reflecting the latest in chemical research and data.
Strategy Settings
Precursor scoring | Relevance Heuristic |
---|---|
Min. plausibility | 0.01 |
Model | Template_relevance |
Template Set | Pistachio/Bkms_metabolic/Pistachio_ringbreaker/Reaxys/Reaxys_biocatalysis |
Top-N result to add to graph | 6 |
Feasible Synthetic Routes
Q1: How do cyclopentylurea derivatives interact with their targets in the context of cholesterol ester transfer protein (CETP) inhibition? What are the downstream effects of this inhibition?
A1: While the provided abstracts don't delve into the specific binding interactions of this compound derivatives with CETP, research [] indicates that these compounds act as CETP inhibitors. CETP facilitates the transfer of cholesteryl esters from high-density lipoprotein (HDL) to low-density lipoprotein (LDL). Inhibiting CETP can lead to increased HDL levels and decreased LDL levels, potentially offering benefits for cardiovascular health.
Q2: Can you provide examples of how the structure of this compound derivatives has been modified to influence their activity as CETP inhibitors?
A2: Research [] showcases the structure-activity relationship (SAR) exploration of diphenylpyridylethanamine (DPPE) derivatives containing a this compound moiety. Replacing a labile ester group in the initial lead compound with amide and urea functionalities led to improved stability. Further optimization involved modifications at the N-terminus of the DPPE scaffold, resulting in compounds like 20 with enhanced pharmacokinetic properties and robust efficacy in preclinical models.
Q3: How have this compound derivatives been utilized in chiral separation applications? What characteristics make them suitable for this purpose?
A3: Research [] highlights the synthesis and application of chitosan-based chiral stationary phases (CSPs) functionalized with this compound and 3,5-dimethylphenylcarbamate groups for enantiomeric separation. These CSPs demonstrated effective enantioseparation capabilities comparable to established cellulose-based CSPs. Importantly, the this compound derivatives exhibited good tolerance to various organic solvents, expanding the range of mobile phase choices for HPLC-based enantiomeric separations. This tolerance stems from the controlled swelling properties of the chitosan derivatives in different solvents.
Disclaimer and Information on In-Vitro Research Products
Please be aware that all articles and product information presented on BenchChem are intended solely for informational purposes. The products available for purchase on BenchChem are specifically designed for in-vitro studies, which are conducted outside of living organisms. In-vitro studies, derived from the Latin term "in glass," involve experiments performed in controlled laboratory settings using cells or tissues. It is important to note that these products are not categorized as medicines or drugs, and they have not received approval from the FDA for the prevention, treatment, or cure of any medical condition, ailment, or disease. We must emphasize that any form of bodily introduction of these products into humans or animals is strictly prohibited by law. It is essential to adhere to these guidelines to ensure compliance with legal and ethical standards in research and experimentation.