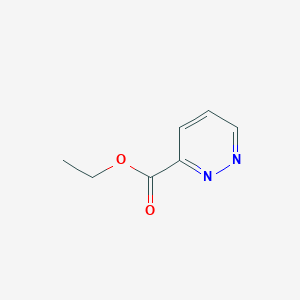
Ethyl pyridazine-3-carboxylate
Overview
Description
Ethyl pyridazine-3-carboxylate (CAS: 1126-10-9) is a heterocyclic compound with the molecular formula C₇H₈N₂O₂ and a purity of 97% . Structurally, it consists of a pyridazine ring substituted with an ester group at the 3-position. This compound serves as a versatile intermediate in pharmaceutical synthesis and coordination chemistry. For instance, it forms monomeric complexes with lithium(I), where the pyridazine-3-carboxylate ligand chelates the metal ion via N,O-coordination, creating a distorted tetrahedral geometry . Such coordination properties highlight its utility in inorganic chemistry and materials science.
Preparation Methods
Esterification of Pyridazine-3-Carboxylic Acid
Acid-Catalyzed Esterification
The most straightforward route involves reacting pyridazine-3-carboxylic acid with ethanol under acidic conditions. Sulfuric acid (H₂SO₄) or hydrochloric acid (HCl) catalyzes the reaction, typically conducted under reflux (78–90°C) for 6–12 hours .
Reaction Mechanism:
Optimization Data:
Parameter | Optimal Range | Yield (%) | Source |
---|---|---|---|
Temperature | 80–90°C | 65–75 | |
Catalyst (H₂SO₄) | 5–10% w/w | 70 | |
Reaction Time | 8–10 hours | 68 |
This method prioritizes simplicity but faces limitations in water removal, often requiring Dean-Stark traps or molecular sieves to shift equilibrium toward ester formation .
Acyl Chloride Route
Pyridazine-3-carbonyl chloride, generated via thionyl chloride (SOCl₂) or oxalyl chloride, reacts with ethanol at 0–25°C to yield the ester. This method avoids equilibrium issues but necessitates strict anhydrous conditions.
Procedure:
-
Acyl Chloride Formation:
-
Esterification:
Advantages:
Cyclization Reactions Involving Ethyl 2-Chloro-3-Oxopropanoate
Cyclization with Halogenated Pyridazines
Industrial-scale synthesis often employs cyclization strategies. For example, 4-bromo-6-chloropyridazin-3-amine reacts with ethyl 2-chloro-3-oxopropanoate in ethanol at 80°C for 16 hours, forming imidazo[1,2-b]pyridazine intermediates . While this method targets analogs, it highlights scalable approaches adaptable to this compound.
Key Data from Analogous Reactions:
Negishi Coupling for Functionalized Derivatives
Palladium-catalyzed cross-coupling introduces substituents post-cyclization. For instance, vinyl groups are added via Negishi coupling, though this applies to derivatives like ethyl 6-vinylimidazo[1,2-b]pyridazine-3-carboxylate.
Optimization of Reaction Conditions
Solvent and Temperature Effects
Ethanol is preferred for esterification due to its dual role as solvent and reactant. Elevated temperatures (80–90°C) improve kinetics but risk side reactions like dehydration .
Catalytic Systems
-
Acid Catalysts: H₂SO₄ outperforms HCl in esterification due to stronger protonation capability .
-
Base Catalysts: Sodium ethoxide (NaOEt) accelerates transesterification but is less common .
Purification and Characterization
Chromatographic Purification
Silica gel chromatography (ethyl acetate/petroleum ether, 10–20% v/v) effectively isolates the product, albeit with moderate recovery (50–60%) .
Spectroscopic Analysis
-
¹H NMR: Ethyl group signals (δ 1.44 ppm, triplet; δ 4.47 ppm, quartet) and aromatic protons (δ 7.38–8.38 ppm) .
-
LC-MS: Molecular ion peak at m/z 153 [M+H]⁺ confirms molecular weight .
Industrial-Scale Production Considerations
Continuous Flow Chemistry
Flow reactors enhance reproducibility and scalability, reducing reaction times by 30–50% compared to batch processes.
Cost-Efficiency Metrics
Factor | Batch Process | Flow Process |
---|---|---|
Ethanol Consumption | 2.5 L/kg product | 1.8 L/kg product |
Energy Cost | $120/kg | $85/kg |
Chemical Reactions Analysis
Nucleophilic Substitution Reactions
The pyridazine ring undergoes regioselective substitution at electron-deficient positions. Key examples include:
Chlorine Replacement
Ethyl 6-chloro-pyridazine-3-carboxylate reacts with nucleophiles like morpholine or sodium azide under mild conditions:
These reactions highlight the susceptibility of the 6-position to nucleophilic attack due to electron withdrawal by the ester group.
Cyclization and Ring Formation
The ester group facilitates cyclization to form fused heterocycles:
Triazolo-Pyridazine Formation
Reaction with hydrazine derivatives promotes cyclization into triazolo[4,3-b]pyridazine systems:
textEthyl pyridazine-3-carboxylate + Hydrazine hydrate → Ethyl [1,2,4]triazolo[4,3-b]pyridazine-3-carboxylate
Conditions: Acetic acid, reflux. This pathway is critical for generating bioactive derivatives.
Ester Functionalization
The ethyl ester undergoes hydrolysis, amidation, and transesterification:
Hydrolysis
-
Acidic Hydrolysis : Concentrated HCl in ethanol yields pyridazine-3-carboxylic acid.
-
Basic Hydrolysis : NaOH in aqueous ethanol produces the sodium salt, which acidifies to the free acid .
Amidation
Primary amines react with the ester under reflux to form carboxamides:
textThis compound + R-NH₂ → Pyridazine-3-carboxamide
Yields depend on steric and electronic effects of the amine .
Multi-Component Reactions
This compound participates in regioselective one-pot syntheses:
Three-Component Coupling
Arylglyoxals, ethyl butyrylacetate, and hydrazine hydrate in water yield ethyl 6-aryl-3-propylpyridazine-4-carboxylates :
textArylglyoxal + Ethyl butyrylacetate + Hydrazine → Ethyl 6-aryl-3-propylpyridazine-4-carboxylate
Key features:
-
Room-temperature reaction in water.
-
Regioselectivity driven by electronic effects of the carbonyl groups .
Oxidation
-
Side-Chain Oxidation : KMnO₄ oxidizes the ethyl group to a carboxylic acid.
-
Ring Oxidation : Ozone or peroxides modify the pyridazine ring, though products are less studied.
Reduction
Catalytic hydrogenation (H₂/Pd-C) reduces the pyridazine ring to a piperazine derivative, altering electronic properties.
Data Table: Biological Activity of Key Derivatives
Scientific Research Applications
Ethyl pyridazine-3-carboxylate is primarily utilized in the synthesis of various pharmaceuticals. Its derivatives have been explored for their potential as anti-inflammatory and anti-cancer agents.
Case Study: Anti-Cancer Activity
Research has demonstrated that derivatives of this compound exhibit cytotoxic effects on cancer cell lines. For instance, modifications to the carboxylate group can enhance the compound's potency against specific tumor types, as shown in studies involving breast cancer cell lines.
Agricultural Chemistry
In agricultural applications, this compound serves as an important building block for developing herbicides and fungicides. Its derivatives can provide selective control over unwanted plant growth while minimizing damage to crops.
Case Study: Herbicidal Activity
A study evaluated the herbicidal efficacy of synthesized this compound derivatives against a range of common weeds. The results indicated that certain derivatives could inhibit weed growth effectively at low concentrations, suggesting their potential use in sustainable agriculture.
Material Science
This compound is also being investigated for its potential applications in material science, particularly in the synthesis of polymers and advanced materials.
Case Study: Polymer Synthesis
Recent research has focused on using this compound as a monomer in polymerization reactions. The resulting polymers exhibited enhanced thermal stability and mechanical properties, making them suitable for various industrial applications.
Synthetic Applications
The compound is widely used as an intermediate in organic synthesis. It can undergo various reactions, including:
- Alkylation : Reacts with alkyl halides to form N-alkylated derivatives.
- Acylation : Can be acylated using acyl chlorides to form amides.
- Reduction : The ketone group can be reduced to an alcohol.
These reactions highlight its versatility as a synthetic building block in organic chemistry.
Summary Table of Applications
Application Area | Specific Use | Example Findings |
---|---|---|
Medicinal Chemistry | Anti-cancer agents | Effective against breast cancer cells |
Agricultural Chemistry | Herbicides and fungicides | Selective weed control |
Material Science | Polymer synthesis | Enhanced thermal stability |
Synthetic Applications | Intermediate for organic synthesis | Versatile reactions (alkylation, acylation) |
Mechanism of Action
The mechanism of action of ethyl pyridazine-3-carboxylate and its derivatives often involves interaction with specific molecular targets, such as enzymes or receptors. For example, some derivatives may inhibit enzymes involved in inflammatory pathways, thereby exerting anti-inflammatory effects. The exact mechanism can vary depending on the specific derivative and its target.
Comparison with Similar Compounds
Ethyl pyridazine-3-carboxylate derivatives are modified via substitutions or ring fusion to enhance biological activity or physicochemical properties. Below is a systematic comparison:
Chloro- and Trifluoromethyl-Substituted Derivatives
Ethyl 6-Chloro-5-(Trifluoromethyl)pyridazine-3-Carboxylate (Compound 6)
- Molecular Formula : C₈H₆ClF₃N₂O₂
- Molecular Weight : 254.59
- Key Data : Melting point 147–148°C; ¹H NMR (CDCl₃): δ 1.35 (t, CH₃), 8.18 (s, CH-pyridazine) .
- Applications : Exhibits anticancer activity in preclinical studies due to the electron-withdrawing trifluoromethyl group enhancing metabolic stability .
Ethyl 4,6-Dichloropyridazine-3-Carboxylate
- Molecular Formula : C₇H₆Cl₂N₂O₂
- Molecular Weight : 221.04
- Applications : A key intermediate in synthesizing kinase inhibitors. Reactivity at the 4- and 6-positions allows selective amination or alkylation .
Comparison : The trifluoromethyl group in Compound 6 improves lipophilicity compared to the dichloro derivative, favoring blood-brain barrier penetration in anticancer applications.
Imidazo-Fused Derivatives
Ethyl 6-Chloro-2-Methylimidazo[1,2-b]pyridazine-3-Carboxylate
- Molecular Formula : C₁₀H₁₀ClN₃O₂
- Molecular Weight : 239.66
- Key Data : CAS 14714-18-2; ChemSpider ID 13204237 .
- Applications : The fused imidazole ring enhances binding to ATP pockets in kinases, making it a candidate for targeted therapies .
Comparison : Ring fusion increases molecular weight by ~32% compared to the parent compound, improving target specificity but reducing solubility.
Fluoro-Substituted Derivatives
Ethyl 7-Chloro-6-Fluoro-1,4-Dihydro-4-Oxopyrido[2,3-c]pyridazine-3-Carboxylate
- Molecular Formula : C₁₀H₇ClFN₃O₃
- Key Data : Antibacterial activity against E. coli (MIC: 50 µg/mL) .
- Mechanism : The fluorine atom increases electronegativity, enhancing interactions with bacterial DNA gyrase .
Comparison: Fluorine substitution confers broader antibacterial activity compared to non-halogenated analogs.
Pyrrolo-Fused Derivatives
Ethyl 4-Oxo-1H-Pyrrolo[1,2-b]pyridazine-3-Carboxylate
- Molecular Formula : C₁₀H₁₀N₂O₃
- Molecular Weight : 206.20
- Key Data : CAS 156335-37-4; used in synthesizing prostaglandin receptor antagonists .
- Structural Impact : The fused pyrrole ring introduces a planar conformation, favoring π-π stacking in receptor binding.
Comparison: The additional ring reduces metabolic clearance compared to non-fused derivatives.
Amino- and Methyl-Substituted Derivatives
Ethyl 6-(Ethylamino)pyridazine-3-Carboxylate
- Molecular Formula : C₈H₁₂N₃O₂
- Key Data : CAS 1823915-03-2; used in kinase inhibitor synthesis .
- Solubility: The ethylamino group improves water solubility via hydrogen bonding.
Ethyl 6-Methylpyridazine-3-Carboxylate
- Molecular Formula : C₈H₁₀N₂O₂
- Molecular Weight : 166.18
- Applications : Simpler structure for probing structure-activity relationships .
Comparison: Amino groups enhance solubility, while methyl groups increase steric hindrance, affecting binding kinetics.
Biological Activity
Ethyl pyridazine-3-carboxylate is a compound that has garnered interest in the field of medicinal chemistry due to its potential biological activities. This article delves into its biological properties, mechanisms of action, and relevant research findings.
Chemical Structure and Properties
This compound is characterized by its pyridazine ring, which is a six-membered heterocyclic compound containing two nitrogen atoms. Its molecular formula is , and it has a molecular weight of approximately 166.18 g/mol. The presence of the carboxylate group enhances its reactivity and potential interactions with biological targets.
Biological Activities
1. Antimicrobial Activity
this compound has been studied for its antimicrobial properties. Research indicates that compounds with similar structures exhibit activity against various pathogens, including bacteria and fungi. For instance, derivatives of pyridazine have shown significant inhibition against Gram-positive and Gram-negative bacteria, suggesting potential as an antibiotic agent .
2. Anticancer Properties
The compound's anticancer activity has been highlighted in several studies. A series of 3,6-disubstituted pyridazines, including ethyl pyridazine derivatives, were evaluated for their effects on cancer cell lines such as MDA-MB-231 (breast cancer) and SKOV-3 (ovarian cancer). Some derivatives demonstrated potent cytotoxicity and induced apoptosis in these cell lines, indicating that this compound may serve as a scaffold for developing anticancer drugs .
3. Anti-inflammatory Effects
this compound is also being explored for its anti-inflammatory properties. Compounds in this class have been shown to inhibit pathways associated with inflammation, potentially offering therapeutic benefits in conditions characterized by chronic inflammation .
The biological effects of this compound are mediated through various mechanisms:
- Enzyme Inhibition: The compound may interact with specific enzymes or receptors, modulating their activity. For example, it could inhibit kinases involved in cancer cell proliferation or inflammatory responses .
- Cell Cycle Arrest: Some studies suggest that ethyl pyridazine derivatives can induce cell cycle arrest in cancer cells, preventing their proliferation and leading to increased apoptosis .
Table 1: Summary of Biological Activities
Activity Type | Findings | Reference |
---|---|---|
Antimicrobial | Effective against various bacterial strains | |
Anticancer | Induced apoptosis in breast and ovarian cancer cells | |
Anti-inflammatory | Inhibited inflammatory pathways |
Case Study: Anticancer Activity
In a study focusing on the synthesis and evaluation of 3,6-disubstituted pyridazines, ethyl pyridazine derivatives were tested against human cancer cell lines. The results indicated that certain derivatives exhibited IC50 values in the low micromolar range, demonstrating significant anticancer efficacy compared to standard treatments .
Q & A
Basic Research Questions
Q. What are the standard synthetic routes for Ethyl pyridazine-3-carboxylate, and how can reaction conditions be optimized for yield and purity?
this compound is commonly synthesized via esterification or substitution reactions. For example, phosphorylation chloride (POCl₃) under reflux conditions is effective for introducing chlorine substituents, achieving yields up to 95% (e.g., in the synthesis of ethyl 3-chloro-5,6-diarylpyridazine-4-carboxylates) . Optimization strategies include:
- Solvent selection : Polar aprotic solvents like DMF enhance reaction efficiency, as seen in cesium carbonate-mediated alkylation steps .
- Temperature control : Reflux conditions (e.g., 80°C for 1–4 hours) improve reaction rates while minimizing side products .
- Purification : Silica gel chromatography (0–80% ethyl acetate/hexane gradients) effectively isolates target compounds .
Q. Which spectroscopic and chromatographic techniques are critical for characterizing this compound derivatives?
Key methods include:
- LCMS : Used to confirm molecular ion peaks (e.g., m/z 657 [M+H]+ for alkylated derivatives) and monitor reaction progress .
- ¹H NMR : Characterizes substitution patterns, such as ethyl ester protons (δ ~4.3 ppm, quartet) and aromatic protons in diaryl derivatives .
- HPLC : Retention time analysis (e.g., 1.05–1.33 minutes under SQD-FA05 conditions) ensures purity .
- IR spectroscopy : Identifies carbonyl stretches (1735 cm⁻¹ for ester groups) and halogen substituents (e.g., C–Cl at 761 cm⁻¹) .
Q. How are common derivatives like halogenated or hydroxylated analogs synthesized from this compound?
- Halogenation : POCl₃ or PCl₃ introduces chlorine at the pyridazine ring, as demonstrated in the synthesis of 3-chloro derivatives .
- Hydroxylation : Alkaline hydrolysis of the ester group yields carboxylic acid intermediates, which can be further functionalized .
- Alkylation : Cesium carbonate in DMF facilitates N-alkylation, as seen in the preparation of 1-pentyl derivatives .
Advanced Research Questions
Q. What mechanistic insights explain side product formation during alkylation or esterification of this compound?
Side products often arise from:
- Over-alkylation : Excess alkylating agents lead to di- or tri-substituted byproducts. Monitoring reaction time and stoichiometry mitigates this .
- Ester hydrolysis : Trace moisture in polar solvents hydrolyzes the ethyl ester to carboxylic acid. Anhydrous conditions (e.g., molecular sieves) are critical .
- Coordination effects : In metal-catalyzed reactions, pyridazine nitrogen can chelate metals, altering reactivity. For example, Pb(II) coordination stabilizes polymeric structures .
Q. How can crystallographic data resolve contradictions in reported molecular geometries of pyridazine-3-carboxylate complexes?
Single-crystal X-ray diffraction reveals:
- Coordination modes : Pyridazine-3-carboxylate ligands adopt bidentate (N,O) or bridging configurations in Pb(II) complexes, forming catenated ribbons .
- Monomeric vs. polymeric structures : Li(I) complexes with pyridazine-3-carboxylate are monomeric, contrasting with polymeric patterns in pyrazine analogs .
- Hydrogen bonding : C–H⋯O interactions stabilize supramolecular assemblies, impacting solubility and reactivity .
Q. What computational approaches are used to predict the reactivity of this compound in drug discovery pipelines?
- DFT calculations : Model electrophilic aromatic substitution (e.g., halogenation) by analyzing frontier molecular orbitals (HOMO/LUMO) .
- Molecular docking : Predict binding affinities of derivatives to target proteins (e.g., kinase inhibitors) based on steric and electronic complementarity .
- ADMET profiling : Tools like SwissADME assess pharmacokinetic properties (e.g., GI absorption, BBB permeability) to prioritize candidates .
Q. How do solvent polarity and additives influence regioselectivity in multi-step functionalization of this compound?
- Polar solvents (DMF, DMSO) : Stabilize charged intermediates, favoring nucleophilic substitution at the pyridazine ring .
- Additives (Cs₂CO₃, K₂CO₃) : Deprotonate acidic N–H sites, directing alkylation to specific positions (e.g., 1-pentyl substitution in methyl esters) .
- Microwave-assisted synthesis : Enhances regioselectivity by reducing reaction times and thermal degradation .
Properties
IUPAC Name |
ethyl pyridazine-3-carboxylate | |
---|---|---|
Source | PubChem | |
URL | https://pubchem.ncbi.nlm.nih.gov | |
Description | Data deposited in or computed by PubChem | |
InChI |
InChI=1S/C7H8N2O2/c1-2-11-7(10)6-4-3-5-8-9-6/h3-5H,2H2,1H3 | |
Source | PubChem | |
URL | https://pubchem.ncbi.nlm.nih.gov | |
Description | Data deposited in or computed by PubChem | |
InChI Key |
LLLVOBWNRQYTKR-UHFFFAOYSA-N | |
Source | PubChem | |
URL | https://pubchem.ncbi.nlm.nih.gov | |
Description | Data deposited in or computed by PubChem | |
Canonical SMILES |
CCOC(=O)C1=NN=CC=C1 | |
Source | PubChem | |
URL | https://pubchem.ncbi.nlm.nih.gov | |
Description | Data deposited in or computed by PubChem | |
Molecular Formula |
C7H8N2O2 | |
Source | PubChem | |
URL | https://pubchem.ncbi.nlm.nih.gov | |
Description | Data deposited in or computed by PubChem | |
DSSTOX Substance ID |
DTXSID50560467 | |
Record name | Ethyl pyridazine-3-carboxylate | |
Source | EPA DSSTox | |
URL | https://comptox.epa.gov/dashboard/DTXSID50560467 | |
Description | DSSTox provides a high quality public chemistry resource for supporting improved predictive toxicology. | |
Molecular Weight |
152.15 g/mol | |
Source | PubChem | |
URL | https://pubchem.ncbi.nlm.nih.gov | |
Description | Data deposited in or computed by PubChem | |
CAS No. |
1126-10-9 | |
Record name | Ethyl pyridazine-3-carboxylate | |
Source | EPA DSSTox | |
URL | https://comptox.epa.gov/dashboard/DTXSID50560467 | |
Description | DSSTox provides a high quality public chemistry resource for supporting improved predictive toxicology. | |
Retrosynthesis Analysis
AI-Powered Synthesis Planning: Our tool employs the Template_relevance Pistachio, Template_relevance Bkms_metabolic, Template_relevance Pistachio_ringbreaker, Template_relevance Reaxys, Template_relevance Reaxys_biocatalysis model, leveraging a vast database of chemical reactions to predict feasible synthetic routes.
One-Step Synthesis Focus: Specifically designed for one-step synthesis, it provides concise and direct routes for your target compounds, streamlining the synthesis process.
Accurate Predictions: Utilizing the extensive PISTACHIO, BKMS_METABOLIC, PISTACHIO_RINGBREAKER, REAXYS, REAXYS_BIOCATALYSIS database, our tool offers high-accuracy predictions, reflecting the latest in chemical research and data.
Strategy Settings
Precursor scoring | Relevance Heuristic |
---|---|
Min. plausibility | 0.01 |
Model | Template_relevance |
Template Set | Pistachio/Bkms_metabolic/Pistachio_ringbreaker/Reaxys/Reaxys_biocatalysis |
Top-N result to add to graph | 6 |
Feasible Synthetic Routes
Disclaimer and Information on In-Vitro Research Products
Please be aware that all articles and product information presented on BenchChem are intended solely for informational purposes. The products available for purchase on BenchChem are specifically designed for in-vitro studies, which are conducted outside of living organisms. In-vitro studies, derived from the Latin term "in glass," involve experiments performed in controlled laboratory settings using cells or tissues. It is important to note that these products are not categorized as medicines or drugs, and they have not received approval from the FDA for the prevention, treatment, or cure of any medical condition, ailment, or disease. We must emphasize that any form of bodily introduction of these products into humans or animals is strictly prohibited by law. It is essential to adhere to these guidelines to ensure compliance with legal and ethical standards in research and experimentation.