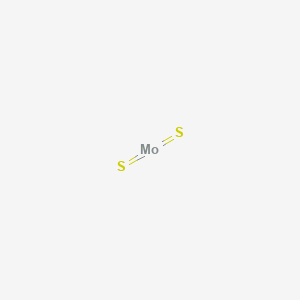
Molybdenum disulfide
Overview
Description
Molybdenum disulfide is an inorganic compound composed of molybdenum and sulfur, with the chemical formula MoS₂. It belongs to the class of transition metal dichalcogenides and is characterized by its silvery black appearance. This compound occurs naturally as the mineral molybdenite, which is the principal ore for molybdenum. This compound is known for its low friction and robustness, making it widely used as a dry lubricant .
Mechanism of Action
Target of Action
Molybdenum disulfide (MoS2) is an inorganic compound composed of molybdenum and sulfur . It is classified as a transition metal dichalcogenide . The primary targets of MoS2 are the surfaces it interacts with, particularly in its role as a dry lubricant . It also has potential as a semiconductor analogue of graphene .
Mode of Action
MoS2 interacts with its targets through its unique layered structure, where a plane of molybdenum atoms is sandwiched by planes of sulfide ions . This structure allows for easy sliding of MoS2 lamellae, leading to its low friction and robustness as a lubricant . In addition, MoS2 can facilitate electron transfer during electrochemical reactions, leading to higher sensitivity and lower detection limits in sensing applications .
Pharmacokinetics
It has been developed for medical uses due to its excellent medically beneficial characteristics . More research is needed to fully understand the ADME properties of MoS2 and their impact on bioavailability.
Result of Action
The result of MoS2’s action depends on its application. As a lubricant, it reduces friction between surfaces . As a semiconductor, it has potential for use in nano-electronic applications . In biological applications, it can influence cell proliferation, adhesion, and immunomodulation .
Action Environment
The action of MoS2 can be influenced by environmental factors. For example, its lubricating properties can be affected by the presence of other substances or changes in temperature . In addition, the properties of MoS2 can change depending on whether it is in its bulk form or in two-dimensional forms .
Biochemical Analysis
Biochemical Properties
Molybdenum disulfide has been found to interact with various biomolecules. For instance, it has been used in the design of a multilayer nanoprobe for ultrasensitive analysis of microRNA-21 . The nature of these interactions is largely due to the unique nanostructures and physical/chemical properties of this compound .
Cellular Effects
The effects of this compound on cells and cellular processes are diverse. It has been shown to have low cytotoxicity and high biocompatibility, making it suitable for biological and biomedical applications .
Molecular Mechanism
At the molecular level, this compound exerts its effects through various mechanisms. These include binding interactions with biomolecules, potential enzyme inhibition or activation, and changes in gene expression
Temporal Effects in Laboratory Settings
In laboratory settings, the effects of this compound can change over time. Information on its stability, degradation, and long-term effects on cellular function is still being studied. Its good electronic, optical, and catalytic properties suggest potential for long-term stability .
Dosage Effects in Animal Models
The effects of this compound can vary with different dosages in animal models. While specific threshold effects and potential toxic or adverse effects at high doses are still being researched, its low cytotoxicity and high biocompatibility suggest a favorable safety profile .
Metabolic Pathways
This compound may be involved in various metabolic pathways. It could potentially interact with enzymes or cofactors, and may have effects on metabolic flux or metabolite levels
Transport and Distribution
The transport and distribution of this compound within cells and tissues is a complex process that is still being studied. It could potentially interact with transporters or binding proteins, and may have effects on its localization or accumulation .
Subcellular Localization
The subcellular localization of this compound and its effects on activity or function are areas of active research. It could potentially be directed to specific compartments or organelles through targeting signals or post-translational modifications .
Preparation Methods
Synthetic Routes and Reaction Conditions: Molybdenum disulfide can be synthesized through various methods, including chemical vapor deposition, hydrothermal synthesis, and liquid-phase exfoliation. One common method involves the thermal treatment of molybdenum compounds with hydrogen sulfide or elemental sulfur. For instance, molybdenum pentachloride can react with hydrogen sulfide to produce this compound .
Industrial Production Methods: Industrially, this compound is produced by processing molybdenite ore through flotation to obtain relatively pure this compound. The main contaminant in this process is carbon. Another method involves the thermal treatment of molybdenum compounds with hydrogen sulfide or elemental sulfur .
Chemical Reactions Analysis
Types of Reactions: Molybdenum disulfide undergoes various chemical reactions, including oxidation, reduction, and intercalation. It is relatively unreactive and is unaffected by dilute acids and oxygen .
Common Reagents and Conditions:
Oxidation: this compound can be oxidized using strong oxidizing agents such as nitric acid or hydrogen peroxide.
Reduction: It can be reduced using hydrogen gas at high temperatures.
Intercalation: Alkali metals can intercalate into the layers of this compound, altering its electronic properties.
Major Products: The major products formed from these reactions include molybdenum trioxide (from oxidation) and reduced molybdenum compounds (from reduction) .
Scientific Research Applications
Molybdenum disulfide has a wide range of applications in scientific research due to its unique properties:
Comparison with Similar Compounds
Molybdenum disulfide is often compared with other transition metal dichalcogenides such as tungsten disulfide and molybdenum diselenide. These compounds share similar layered structures and properties but differ in their electronic and mechanical characteristics:
Tungsten Disulfide (WS₂): Similar to this compound, tungsten disulfide is used as a lubricant and has a higher thermal stability.
Molybdenum Diselenide (MoSe₂): This compound has a similar structure but exhibits different electronic properties due to the presence of selenium instead of sulfur.
This compound stands out due to its unique combination of low friction, high wear resistance, and semiconductor properties, making it a versatile material for various applications .
Properties
IUPAC Name |
bis(sulfanylidene)molybdenum | |
---|---|---|
Source | PubChem | |
URL | https://pubchem.ncbi.nlm.nih.gov | |
Description | Data deposited in or computed by PubChem | |
InChI |
InChI=1S/Mo.2S | |
Source | PubChem | |
URL | https://pubchem.ncbi.nlm.nih.gov | |
Description | Data deposited in or computed by PubChem | |
InChI Key |
CWQXQMHSOZUFJS-UHFFFAOYSA-N | |
Source | PubChem | |
URL | https://pubchem.ncbi.nlm.nih.gov | |
Description | Data deposited in or computed by PubChem | |
Canonical SMILES |
S=[Mo]=S | |
Source | PubChem | |
URL | https://pubchem.ncbi.nlm.nih.gov | |
Description | Data deposited in or computed by PubChem | |
Molecular Formula |
MoS2 | |
Record name | Molybdenum disulfide | |
Source | Wikipedia | |
URL | https://en.wikipedia.org/wiki/Molybdenum_disulfide | |
Description | Chemical information link to Wikipedia. | |
Source | PubChem | |
URL | https://pubchem.ncbi.nlm.nih.gov | |
Description | Data deposited in or computed by PubChem | |
DSSTOX Substance ID |
DTXSID201318098 | |
Record name | Molybdenite | |
Source | EPA DSSTox | |
URL | https://comptox.epa.gov/dashboard/DTXSID201318098 | |
Description | DSSTox provides a high quality public chemistry resource for supporting improved predictive toxicology. | |
Molecular Weight |
160.1 g/mol | |
Source | PubChem | |
URL | https://pubchem.ncbi.nlm.nih.gov | |
Description | Data deposited in or computed by PubChem | |
Physical Description |
Dry Powder, Water or Solvent Wet Solid, Liquid; Liquid; Other Solid; Pellets or Large Crystals, Lustrous lead-grey powder; Black and lustrous when artificially prepared; Insoluble in water; [Merck Index] Black odorless powder; Insoluble in water; [MSDSonline] | |
Record name | Molybdenum sulfide (MoS2) | |
Source | EPA Chemicals under the TSCA | |
URL | https://www.epa.gov/chemicals-under-tsca | |
Description | EPA Chemicals under the Toxic Substances Control Act (TSCA) collection contains information on chemicals and their regulations under TSCA, including non-confidential content from the TSCA Chemical Substance Inventory and Chemical Data Reporting. | |
Record name | Molybdenum disulfide | |
Source | Haz-Map, Information on Hazardous Chemicals and Occupational Diseases | |
URL | https://haz-map.com/Agents/8284 | |
Description | Haz-Map® is an occupational health database designed for health and safety professionals and for consumers seeking information about the adverse effects of workplace exposures to chemical and biological agents. | |
Explanation | Copyright (c) 2022 Haz-Map(R). All rights reserved. Unless otherwise indicated, all materials from Haz-Map are copyrighted by Haz-Map(R). No part of these materials, either text or image may be used for any purpose other than for personal use. Therefore, reproduction, modification, storage in a retrieval system or retransmission, in any form or by any means, electronic, mechanical or otherwise, for reasons other than personal use, is strictly prohibited without prior written permission. | |
Boiling Point |
450 °C, sublimes | |
Record name | MOLYBDENUM DISULFIDE | |
Source | Hazardous Substances Data Bank (HSDB) | |
URL | https://pubchem.ncbi.nlm.nih.gov/source/hsdb/1660 | |
Description | The Hazardous Substances Data Bank (HSDB) is a toxicology database that focuses on the toxicology of potentially hazardous chemicals. It provides information on human exposure, industrial hygiene, emergency handling procedures, environmental fate, regulatory requirements, nanomaterials, and related areas. The information in HSDB has been assessed by a Scientific Review Panel. | |
Solubility |
INSOL IN WATER OR DIL ACIDS, SOL IN HOT SULFURIC ACID, AQUA REGIA, NITRIC ACID | |
Record name | MOLYBDENUM DISULFIDE | |
Source | Hazardous Substances Data Bank (HSDB) | |
URL | https://pubchem.ncbi.nlm.nih.gov/source/hsdb/1660 | |
Description | The Hazardous Substances Data Bank (HSDB) is a toxicology database that focuses on the toxicology of potentially hazardous chemicals. It provides information on human exposure, industrial hygiene, emergency handling procedures, environmental fate, regulatory requirements, nanomaterials, and related areas. The information in HSDB has been assessed by a Scientific Review Panel. | |
Density |
5.06 @ 15 °C/15 °C | |
Record name | MOLYBDENUM DISULFIDE | |
Source | Hazardous Substances Data Bank (HSDB) | |
URL | https://pubchem.ncbi.nlm.nih.gov/source/hsdb/1660 | |
Description | The Hazardous Substances Data Bank (HSDB) is a toxicology database that focuses on the toxicology of potentially hazardous chemicals. It provides information on human exposure, industrial hygiene, emergency handling procedures, environmental fate, regulatory requirements, nanomaterials, and related areas. The information in HSDB has been assessed by a Scientific Review Panel. | |
Color/Form |
LEAD-GRAY, LUSTROUS POWDER, Black luster, hexagonal crystals | |
CAS No. |
1309-56-4, 1317-33-5 | |
Record name | Molybdenite | |
Source | CAS Common Chemistry | |
URL | https://commonchemistry.cas.org/detail?cas_rn=1309-56-4 | |
Description | CAS Common Chemistry is an open community resource for accessing chemical information. Nearly 500,000 chemical substances from CAS REGISTRY cover areas of community interest, including common and frequently regulated chemicals, and those relevant to high school and undergraduate chemistry classes. This chemical information, curated by our expert scientists, is provided in alignment with our mission as a division of the American Chemical Society. | |
Explanation | The data from CAS Common Chemistry is provided under a CC-BY-NC 4.0 license, unless otherwise stated. | |
Record name | Molybdenum disulfide | |
Source | CAS Common Chemistry | |
URL | https://commonchemistry.cas.org/detail?cas_rn=1317-33-5 | |
Description | CAS Common Chemistry is an open community resource for accessing chemical information. Nearly 500,000 chemical substances from CAS REGISTRY cover areas of community interest, including common and frequently regulated chemicals, and those relevant to high school and undergraduate chemistry classes. This chemical information, curated by our expert scientists, is provided in alignment with our mission as a division of the American Chemical Society. | |
Explanation | The data from CAS Common Chemistry is provided under a CC-BY-NC 4.0 license, unless otherwise stated. | |
Record name | Molybdenite | |
Source | ChemIDplus | |
URL | https://pubchem.ncbi.nlm.nih.gov/substance/?source=chemidplus&sourceid=0001309564 | |
Description | ChemIDplus is a free, web search system that provides access to the structure and nomenclature authority files used for the identification of chemical substances cited in National Library of Medicine (NLM) databases, including the TOXNET system. | |
Record name | Molybdenum disulfide | |
Source | ChemIDplus | |
URL | https://pubchem.ncbi.nlm.nih.gov/substance/?source=chemidplus&sourceid=0001317335 | |
Description | ChemIDplus is a free, web search system that provides access to the structure and nomenclature authority files used for the identification of chemical substances cited in National Library of Medicine (NLM) databases, including the TOXNET system. | |
Record name | Molybdenum sulfide (MoS2) | |
Source | EPA Chemicals under the TSCA | |
URL | https://www.epa.gov/chemicals-under-tsca | |
Description | EPA Chemicals under the Toxic Substances Control Act (TSCA) collection contains information on chemicals and their regulations under TSCA, including non-confidential content from the TSCA Chemical Substance Inventory and Chemical Data Reporting. | |
Record name | Molybdenite | |
Source | EPA DSSTox | |
URL | https://comptox.epa.gov/dashboard/DTXSID201318098 | |
Description | DSSTox provides a high quality public chemistry resource for supporting improved predictive toxicology. | |
Record name | Molybdenite | |
Source | European Chemicals Agency (ECHA) | |
URL | https://echa.europa.eu/substance-information/-/substanceinfo/100.013.794 | |
Description | The European Chemicals Agency (ECHA) is an agency of the European Union which is the driving force among regulatory authorities in implementing the EU's groundbreaking chemicals legislation for the benefit of human health and the environment as well as for innovation and competitiveness. | |
Explanation | Use of the information, documents and data from the ECHA website is subject to the terms and conditions of this Legal Notice, and subject to other binding limitations provided for under applicable law, the information, documents and data made available on the ECHA website may be reproduced, distributed and/or used, totally or in part, for non-commercial purposes provided that ECHA is acknowledged as the source: "Source: European Chemicals Agency, http://echa.europa.eu/". Such acknowledgement must be included in each copy of the material. ECHA permits and encourages organisations and individuals to create links to the ECHA website under the following cumulative conditions: Links can only be made to webpages that provide a link to the Legal Notice page. | |
Record name | Molybdenum disulphide | |
Source | European Chemicals Agency (ECHA) | |
URL | https://echa.europa.eu/substance-information/-/substanceinfo/100.013.877 | |
Description | The European Chemicals Agency (ECHA) is an agency of the European Union which is the driving force among regulatory authorities in implementing the EU's groundbreaking chemicals legislation for the benefit of human health and the environment as well as for innovation and competitiveness. | |
Explanation | Use of the information, documents and data from the ECHA website is subject to the terms and conditions of this Legal Notice, and subject to other binding limitations provided for under applicable law, the information, documents and data made available on the ECHA website may be reproduced, distributed and/or used, totally or in part, for non-commercial purposes provided that ECHA is acknowledged as the source: "Source: European Chemicals Agency, http://echa.europa.eu/". Such acknowledgement must be included in each copy of the material. ECHA permits and encourages organisations and individuals to create links to the ECHA website under the following cumulative conditions: Links can only be made to webpages that provide a link to the Legal Notice page. | |
Record name | MOLYBDENUM DISULFIDE | |
Source | FDA Global Substance Registration System (GSRS) | |
URL | https://gsrs.ncats.nih.gov/ginas/app/beta/substances/ZC8B4P503V | |
Description | The FDA Global Substance Registration System (GSRS) enables the efficient and accurate exchange of information on what substances are in regulated products. Instead of relying on names, which vary across regulatory domains, countries, and regions, the GSRS knowledge base makes it possible for substances to be defined by standardized, scientific descriptions. | |
Explanation | Unless otherwise noted, the contents of the FDA website (www.fda.gov), both text and graphics, are not copyrighted. They are in the public domain and may be republished, reprinted and otherwise used freely by anyone without the need to obtain permission from FDA. Credit to the U.S. Food and Drug Administration as the source is appreciated but not required. | |
Record name | MOLYBDENUM DISULFIDE | |
Source | Hazardous Substances Data Bank (HSDB) | |
URL | https://pubchem.ncbi.nlm.nih.gov/source/hsdb/1660 | |
Description | The Hazardous Substances Data Bank (HSDB) is a toxicology database that focuses on the toxicology of potentially hazardous chemicals. It provides information on human exposure, industrial hygiene, emergency handling procedures, environmental fate, regulatory requirements, nanomaterials, and related areas. The information in HSDB has been assessed by a Scientific Review Panel. | |
Melting Point |
2375 °C | |
Record name | MOLYBDENUM DISULFIDE | |
Source | Hazardous Substances Data Bank (HSDB) | |
URL | https://pubchem.ncbi.nlm.nih.gov/source/hsdb/1660 | |
Description | The Hazardous Substances Data Bank (HSDB) is a toxicology database that focuses on the toxicology of potentially hazardous chemicals. It provides information on human exposure, industrial hygiene, emergency handling procedures, environmental fate, regulatory requirements, nanomaterials, and related areas. The information in HSDB has been assessed by a Scientific Review Panel. | |
Q1: What is the molecular formula and weight of molybdenum disulfide?
A1: this compound has the molecular formula MoS2. Its molecular weight is 160.07 g/mol.
Q2: How can we characterize the structure of MoS2?
A2: Several techniques are used to characterize MoS2, including:
- X-ray diffraction (XRD): Provides information about the crystal structure and phase purity. [, , , , ]
- Raman Spectroscopy: Identifies characteristic vibrational modes, confirming the presence of MoS2 and providing insights into the number of layers. [, , , ]
- Transmission electron microscopy (TEM): Visualizes the morphology and size of MoS2 nanosheets and allows for the determination of layer numbers. [, , , , ]
- Scanning electron microscopy (SEM): Offers information about the surface morphology and structure of MoS2 materials. [, , , ]
Q3: Can the reoxidation of chemically exfoliated MoS2 be prevented?
A3: While complete prevention of reoxidation is challenging, storing the dispersions under inert atmosphere and minimizing exposure to air can help maintain their properties for a longer duration.
Q4: What are the notable catalytic properties of this compound?
A4: MoS2 exhibits catalytic activity in various reactions, including:
- Hydrodesulfurization: Removal of sulfur from petroleum fractions. [, ]
- Hydrodenitrogenation: Removal of nitrogen from petroleum fractions. []
- Hydrogen evolution reaction (HER): Production of hydrogen from water splitting. [, , ]
- Organic synthesis: Catalysis of various organic reactions, including reduction and heterocycle synthesis. []
Q5: How does the morphology of MoS2 influence its catalytic activity?
A8: The catalytic activity of MoS2 is strongly dependent on its morphology. Studies on MoS2 counter electrodes in dye-sensitized solar cells (DSSCs) have shown that the edge sites of MoS2 are more catalytically active than the basal planes. []
Q6: How can the catalytic activity of MoS2 be enhanced?
A9: Several strategies can enhance the catalytic activity of MoS2, including: * Defect engineering: Introducing defects like vacancies and doping with heteroatoms. [] * Creating hybrid nanostructures: Combining MoS2 with other materials, such as carbon nanotubes or graphene oxide. [, ] * Controlling the morphology and size of MoS2 nanostructures. [, ]
Q7: What are some applications of this compound in energy storage?
A7: MoS2 is a promising electrode material for batteries due to its high theoretical capacity. It has been investigated for use in:
- Lithium-ion batteries: Offering high energy density potential. [, ]
- Sodium-ion batteries: A more sustainable alternative to lithium-ion batteries. []
- Potassium-ion batteries: Exploring new avenues for high-performance energy storage. []
Q8: How does doping affect the performance of MoS2 in lithium-sulfur batteries?
A11: Doping MoS2 with vanadium enhances its performance as a carbon-free sulfur host in lithium-sulfur batteries. The doped material (VMS) exhibits improved sulfur utilization, reduced polysulfide shuttling, and faster redox kinetics, leading to higher capacity and better cycling stability. []
Q9: How is computational chemistry used to understand MoS2?
A12: Computational techniques play a crucial role in: * Investigating reaction mechanisms: Density Functional Theory (DFT) calculations help elucidate the molecular mechanisms involved in the sulfurization of molybdenum trioxide to MoS2. [, ] * Predicting material properties: DFT calculations help predict the electronic structure and optical properties of MoS2. [, , ] * Designing novel MoS2-based materials: Simulations assist in designing and optimizing MoS2-based materials for various applications. [, , , ]
Q10: Can you elaborate on the role of molybdenum oxysulfide rings in MoS2 formation?
A13: DFT calculations have revealed that molybdenum oxysulfide rings are key intermediates in the sulfurization of molybdenum trioxide to MoS2. These rings are formed through a series of nucleophilic addition-elimination reactions with sulfur allotropes. []
Q11: What are some potential applications of MoS2 in electronics?
A11: MoS2 holds promise for:
- Field-effect transistors (FETs): Its semiconducting properties make it suitable for low-power electronics. []
- Sensors: Its sensitivity to environmental changes makes it suitable for sensing applications. [, ]
- Optoelectronic devices: Its unique optical properties make it suitable for applications in light emission and detection. [, , ]
Q12: Are there environmental concerns associated with this compound?
A12: While MoS2 itself is considered relatively benign, the synthesis and disposal of MoS2-based materials should be carefully managed to minimize potential environmental impacts. Research into ecotoxicological effects and sustainable manufacturing practices is ongoing.
Q13: What are the prospects for recycling and waste management of MoS2-based materials?
A13: Developing efficient and environmentally friendly methods for recycling and disposal of MoS2-based materials is crucial for their sustainable use. Research in this area is ongoing, focusing on recovering valuable components and minimizing waste generation.
Q14: What are some of the current research trends in the field of MoS2?
A14: Current research is focused on:
- Defect engineering for enhanced properties: Tailoring the electronic and catalytic properties of MoS2 by controlling defects. [, ]
- Developing novel synthesis methods: Exploring scalable and cost-effective methods for producing high-quality MoS2 materials. [, , , ]
- Expanding applications in diverse fields: Exploring the potential of MoS2 in areas such as energy storage, electronics, catalysis, and biomedical applications. [, , , , ]
Disclaimer and Information on In-Vitro Research Products
Please be aware that all articles and product information presented on BenchChem are intended solely for informational purposes. The products available for purchase on BenchChem are specifically designed for in-vitro studies, which are conducted outside of living organisms. In-vitro studies, derived from the Latin term "in glass," involve experiments performed in controlled laboratory settings using cells or tissues. It is important to note that these products are not categorized as medicines or drugs, and they have not received approval from the FDA for the prevention, treatment, or cure of any medical condition, ailment, or disease. We must emphasize that any form of bodily introduction of these products into humans or animals is strictly prohibited by law. It is essential to adhere to these guidelines to ensure compliance with legal and ethical standards in research and experimentation.